Fitness
Elevated Fibrinogen-to-Albumin Ratio Correlates Stroke | JIR
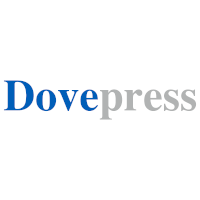
Introduction
Cerebral small vessel disease (CSVD) is a group of cerebrovascular disorders caused by a number of different etiologies affecting mainly small perforating arterioles, capillaries, and small veins. As defined by the updated Standards for Reporting Vascular Changes on Neuroimaging 2 (STRIVE-2), CSVD is characterized by neuroimaging markers, including recent small subcortical infarcts (RSSI), lacune of presumed vascular origin, white matter hyperintensities (WMHs) of presumed vascular origin, enlarged perivascular spaces (EPVS), cerebral microbleeds, cortical superficial siderosis, cortical cerebral microinfarcts, and cerebral atrophy.1,2 Clinically, the recurrence of stroke events is not uncommon among patients with CSVD, with some patients even experiencing frequent recurrences. Previous studies have reported recurrence rates for specific CSVD types, such as lacunar infarction (LI) and cerebral autosomal dominant arteriopathy subcortical infarcts leukoencephalopathy (CADASIL), ranging from 2.7% to 7.3% of patients per year,3–5 and some risk factors have been identified.3,6–9 However, there remains a lack of clarity regarding the proportion and specific risk factors for incident stroke (IS) within a broader CSVD population defined by imaging markers of CVSD.
Longitudinal studies have shown that elevated systemic inflammatory markers present initially can forecast the severity and progression of CSVD. These markers affect the development of CSVD by impacting the permeability of the blood-brain barrier (BBB).10,11 Fibrinogen to Albumin Ratio (FAR), a novel marker of systemic inflammation, has been linked to prognosis in various cancers, such as breast and hepatocellular carcinoma. Furthermore, FAR is associated with recurrence and outcomes after acute ischemic stroke (AIS) and hemorrhagic transformation (HT) following thrombolysis.12–15 Despite these findings, there is a paucity of research on FAR’s role in predicting outcomes in CSVD patients. Our study aims to address this gap by examining the relationship between FAR and the risk of IS and composite vascular events (CVE) in a broader CSVD population.
Methods
Patients Selection
Patients were screened from a hospital based CVSD database in the First Affiliated Hospital of Zhengzhou University from January 2017 to December 2022. The inclusion criteria of the database were as follows: (1) age≥18 years; (2) visible CSVD lesion on brain magnetic resonance imaging (MRI) meeting any of these:1,2,16 1) cerebral WMHs with a Fazekas score≥2; 2) Fazekas=1 with≥2 vascular risk factors (hypertension, hyperlipidemia, diabetes mellitus, obesity and prior vascular events other than stroke, etc).; 3) RSSI (a recent infarction occurring within the past approximately 3 weeks located in the region of a single penetrating artery with a maximum axial diameter of no more than 20 mm and explaining the clinical symptoms).; 4) possible vasculogenic lacunar foci (penetrating arterial distribution 3–15 mm in diameter with cerebrospinal fluid signal); 5) enlarged perivascular space (EPVS, ≤3 mm in diameter along the vascular pathway); and 6) cerebral microbleeds foci (low signal on susceptibility-weighted imaging [SWI], ≤10 mm in diameter, no high signal on T1 and T2-weighted MRI for the corresponding lesion); (3) daily living ability (modified Rankin score≤2). Exclusion criteria: (1) large recent/old infarcts on diffusion weighted imaging (DWI) of the brain (middle cerebral artery region > 1/3, cerebellar hemisphere volume > 1/3, or Alberta Stroke Program Early CT-DWI (ASPECT-DWI) score less than 7); (2) patients with acute intracerebral hemorrhage or large vessel stenosis exceeding 50%; (3) dementia from diagnosed neurodegenerative diseases; (4) non-vascular white matter lesions; (5) history of cerebral vascular malformations, subarachnoid hemorrhage from cerebral aneurysms, or untreated aneurysm (>3mm in diameter); (6) diagnosis of mental disorders according to DSM-V diagnostic criteria; (7) intracranial occupancy, toxicity, metabolic or infectious, and demyelination-related disorders; and (8) suffering from severe organic diseases, such as malignant tumors, with an expected survival time of less than 5 years. In this study, we further excluded: (1) patients with incomplete demographic information, hematologic data, and imaging information; (2) patients who did not sign an informed consent form. The study was conducted with the approval of the Ethics Committee of the First Affiliated Hospital of Zhengzhou University, Ethics Approval No. 2021-KY-1059-002.
Data Collection
We collected basic information (including gender, age, past medical history, smoking history, drinking history, etc)., laboratory information (including routine blood, blood lipids, fasting blood glucose (FBG), glycosylated hemoglobin (HbA1c), liver and kidney function, etc)., and imaging information from each patient. Hypertension was defined as systolic blood pressure (SBP)≥140 mmHg, diastolic blood pressure (DBP)≥90 mmHg or history of antihypertensive medication. Diabetes mellitus was identified as FBG>7.0 mmol/L or HbA1c>6.5% or former use of hypoglycemic drugs. Hyperlipidemia was defined as total cholesterol (TC) >5.0 mmol/L or low-density lipoprotein (LDL) cholesterol >3.62 mmol/L or prior lipid-lowering medication. History of smoking was defined as regular ongoing smoking or abstinence from smoking during the past 6 months. History of drinking was defined as ongoing regular use of alcohol or withdrawal from alcohol use within 6 months. FAR was defined as the ratio of fibrinogen to albumin (ALB).17 For the collection of hematological indices, all patients were tested by blood collection on the morning of the second day after admission, making sure that the patients were in a fasting state and had fasted for not less than 8 hours.
Imaging Assessment
Due to the unavailability of SWI as a routine and obtainable sequence, this study primarily focused on evaluating certain CSVD imaging markers, including lacunar infarction (LI), WMHs, and EPVS from each patient’s images. Conventional sequences included T1 and T2 scans, DWI and apparent diffusion coefficient (ADC), and fluid attenuated inversion recovery (FLAIR) sequences. Patients were examined by Siemens 3.0T superconducting MRI (Prisma, Verio and Skyra), following an imaging protocol that encompassed: Transverse DWI sequence with a 5mm slice thickness, repetition time (TR) of 4600ms, echo time (TE) of 80ms, b values 0 and 1000s/mm², and automated ADC map reconstruction. Transverse and sagittal T1-weighted imaging at 5mm slice thickness with TE of 2.5ms, transverse T2-weighted imaging at 5mm slice thickness with TE of 2.5ms, and transverse T2 FLAIR at 5mm slice thickness with TR of 6500ms and TE of 85ms.
LI was defined as the presence of at least one visible lacunar foci within the brain parenchyma. WMHs was classified into paraventricular and deep WMHs based on their location, whereas high-grade WMHs (HWMH) was defined as a Fazekas score ≥2 for paraventricular or deep WMHs.18 EPVS were classified into centrum semiovale and basal ganglia region based on their locations, while high-grade EPVS (HPVS) was defined as the number of visible EPVS exceeded 10 in the basal ganglia region (counted on the side with the highest number).19 The imaging information was evaluated by two neurologists who were unaware of the patient’s clinical information, and when a disagreement existed, a third neurologist evaluated and ultimately reached a consensus.
Outcomes
In the study, we performed follow-up of CSVD patients by telephone or face-to-face clinic visits at 3, 6, 12, 24 months or over 36 months after discharge from the hospital routinely. All patients were followed from enrollment until the time of the terminal events, the last clinically documented visit, or the end of the last follow-up visit (September 30, 2023). The terminal events we focused on during the follow-up were whether the patient had IS, myocardial infarction (MI), or deaths after discharge from the hospital. The primary outcome of this study was IS (including ischemic or hemorrhagic stroke, lethal or non-lethal). Contacting patients by phone or face-to-face clinic visits, IS was confirmed by querying patients whether they had visited a healthcare facility for any new episode of limb or facial weakness or numbness, or vision, speech, and swallowing dysfunction lasting for more than 24 hours, and whether the diagnosis of stroke (ischemic stroke or cerebral hemorrhage) was already confirmed by a specialized healthcare facility. The secondary outcome was CVE that included the occurrence of IS, MI, and vascular deaths.20 Vascular deaths referred to the deaths due to ischemic stroke, intracranial hemorrhage, MI, heart failure, or other unspecified nonvascular deaths that do not meet the criteria for lethal stroke, MI, or hemorrhage.21
Statistics Analysis
In our study, all analyses and visualizations performed using SPSS (version 26.0) and R (version 4.1.2) software. Missing values for continuous variables were imputed with the mean or median for continuous variables and the plurality method for categorical variables. Continuous variables were expressed as mean (standard deviation) or median (quartiles). Group differences were tested using t-tests or Mann–Whitney U-tests, as appropriate. Categorical variables were presented as number (rate), and differences were assessed using the chi-square test. Univariate and multivariate analyses were performed using the COX proportional risk model. Cumulative probability curves were plotted for the different groups with the Log rank test. Considering competing events, both univariate and multivariate analyses based on the competing risk model were conducted. Nelson-Aalen cumulative risk curves were generated using the cumulative incidence function (CIF), and Fine-Gray’s test assessed significant differences in the risk function between variable groups. The “survminer” package determined the optimal cutoff point for continuous variables with Maximally Selected Rank Statistics (MSRS) identifying appropriate cutoff values. Restricted Cubic Spline (RCS) analysis explored the association between FAR and the risk of stroke recurrence and CVE in CSVD patients. Subgroup analysis of FAR associated with the risk of stroke recurrence and CVE was performed. Two-tailed P-value
Results
Baseline Characteristics
Of the 787 patients initially included in the prospective CSVD database spanning from January 2017 to December 2022, a total of 682 CSVD patients were enrolled in the final analysis after excluding 92 individuals due to insufficient clinical and imaging data, and 13 who declined to provide the informed consent (Figure 1). The patients’ mean age was 61.0±11.1 years, and 260 (38.1%) were female. Further baseline information is presented in Table S1.
Figure 1 Patient screening flow chart. Abbreviation: CSVD, cerebral small vessel disease. |
Outcomes
Over a median follow-up period of 34.0 [interquartile range, 24.0–53.0] months, 32 (4.69%) were lost to follow-up. The primary outcome, IS, occurred in 33 (4.84%, 1.55/100 person-years) patients, comprising 4 cases of intracerebral hemorrhage, 24 cases of ischemic stroke, and 5 cases with unidentified subtypes. Additionally, 4 (0.59%, 0.19/100 person-years) patients had MI, 15 (2.20%, 0.70/100 person-years) died from causes unrelated to stroke, and in total, 37 (5.43%, 1.74/100 person-years) experienced CVE.
Univariate Analysis of Risk Factors for IS and CVE
In the univariate COX regression analysis, higher age (P=0.045), monocyte count (P=0.046), fibrinogen level (P=0.048) and higher FAR (P=0.001) were positively associated with IS in CSVD patients, Conversely, higher ALB (P=0.005) and a higher proportion of LI (P=0.013) were negatively correlated with IS in CSVD patients (Table 1). Additionally, higher age (P=0.005), monocyte count (P=0.013), fibrinogen level (P=0.018), higher FAR (PTable 1).
![]() |
Table 1 Univariate COX Regression Analysis of Risk Factors for Incident Stroke and Composite Vascular Events in Patients with Cerebral Small Vessel Disease |
In the univariate competing risk model, higher fibrinogen level (P=0.025), higher FAR (P=0.002) and a higher proportion of LI (P=0.014) were positively associated with IS. Conversely, higher ALB (P=0.013) was negatively correlated with IS (Table 2). Moreover, higher age (P=0.016), monocyte count (P=0.014), fibrinogen level (P=0.01), higher FAR (PTable 2).
![]() |
Table 2 Univariate Competing Risk Model of Risk Factors for Incident Stroke and Composite Vascular Events in Patients with Cerebral Small Vessel Disease |
Multivariate Analysis of Risk Factors for IS and CVE
In the multivariate COX regression analysis, higher FAR (Hazard Ratio[HR] 1.146; 95% confidence interval [CI] 1.043–1.259; P=0.004) was positively associated with IS in CSVD patients independently after adjusting for age, LI and monocyte count, Meanwhile, a higher FAR (HR1.156; 95% CI 1.063–1.257; P=0.001) were positively correlated with CVE independently after adjusting for age, LI, monocyte count, eGFR and HWMHs (Table 3). Using the “survminer” package, an optimal cutoff value of 8.69 for FAR was obtained (Figure S1). Consequently, FAR > 8.69 was classified as the high group, and FAR ≤ 8.69 as the low group. The cumulative probability curves indicated that the group with FAR > 8.69 in CSVD patients had a significantly elevated risk of IS (P=0.0043) and CVE (P=0.00052) compared to the group with FAR ≤ 8.69. (Figure 2A and B).Furthermore, in the univariate competing risk model, higher FAR was positively associated with IS (HR 1.16; 95% CI 1.06–1.27; P=0.001) after adjusting for LI, and higher FAR was negatively correlated with CVE (HR 1.15; 95% CI 1.05–1.26; P=0.003) after adjusting for age, LI, monocyte count, eGFR, and HWMHs (Table 3). Additionally, Nelson-Aalen cumulative risk curves demonstrated that CSVD patients in the FAR > 8.69 group still had a higher risk of IS (P=0.006) and CVE (PFigure 2C and D).
![]() |
Table 3 Multivariate Analysis of Risk Factors for Incident Stroke and Composite Vascular Events in Patients with Cerebral Small Vessel Disease |
Risk of IS and CVE with FAR Through RCS Analysis
RCS analysis was employed to flexibly model and visualize the relationship between FAR and the risk of IS and CVE in CSVD patients (Figure 3). FAR demonstrated a linear association with the risk of IS (P for non-linearity = 0.7016). Specifically, when FAR > 8.69, the risk of IS increased with the rising FAR (P = 0.0041) (Figure 3A). Similarly, a linear relationship was observed between FAR and the risk of CVE (P for non-linearity = 0.6475). In cases where FAR > 8.69, the risk of CVE increased with the rise of FAR (P = 0.0048) (Figure 3B).
Subgroup Analysis
In subgroup analysis, an interaction was noted between the FAR and gender (P=0.04), age (P=0.005), and history of cerebrovascular disease (P=0.011). In comparison to the low FAR group (FAR≤8.69), the high FAR group (FAR>8.69) was associated with a significantly increased risk of IS among patients aged >60 years (adjusted HR 3.825; 95% CI 1.553–9.425). Similarly, interactions were identified between the FAR and age (P60 years (adjusted HR 3.118; 95% CI 1.257–7.736) compared with the low FAR group (Figure 4).
Comparison of Baseline Information Between FAR>8.69 and FAR≤8.69 Groups
Compared with the FAR≤8.69 group, the FAR >8.69 group had higher age (PTable S1). There was a higher incidence of IS (3.52/100 vs 1.28/100 person-years; rate ratio, 2.764 [95% CI, 1.13–6.158]) and CVE (4.32/100 vs 1.39/100 person-years; rate ratio, 3.118[95% CI, 1.39–6.53]) in the FAR>8.69 group compared with the FAR≤8.69 group.
Discussion
Our study established an independent association between elevated FAR and the risk of IS and CVE in patients with CSVD. Employing 8.69 as the optimal cutoff value, RCS analysis revealed a linear relationship: as FAR exceeded 8.69, the risk of IS and CVE in CSVD patients increased proportionally, with a more pronounced effect observed in individuals aged over 60 years.
In our study, 33 participants experienced IS, with an incidence rate of 4.84% (1.55 per 100 person-years). Previous investigations predominantly focused on specific subsets of CSVD patients, such as LI and CADASIL. In the Secondary Prevention of Small Subcortical Strokes (SPS3) study, the risk of recurrent stroke among patients assigned to receive aspirin alone was 2.7% per year.3 Yasuhiro et al reported an incidence of 4.43 per 100 patient-years in a group of patients with lacunar stroke with single antiplatelet therapy.4 Another study detected incident lacunes in approximately 7.3% of patients per year in a group diagnosed with CADASIL.5 CADASIL, being a distinct subtype, inherently carries a higher risk of recurrence, whether symptomatic or asymptomatic, and can even present with frequent silent acute microinfarcts on imaging.22 Our study indicates a lower IS occurrence rate, potentially attributed to the diverse population, including patients with isolated conditions such as WMHs and EPVS. Moreover, hypertension, diabetes mellitus, and orthostatic hypotension were identified as risk factors for IS in previous studies. However, IS-related risk factors remain to be clarified to avoid the potential exposure to future terminal events in the general CSVD patients. We therefore excluded patients with lesions of macrovascular origin and other causes such as nonvascular lesions, degenerative disorders, metabolic, toxic, and malignant neoplasms to ensure that the overall CSVD population was represented.
In our study, we observed a significant association between FAR, an emerging inflammation indicator, and both IS and CVE in patients with CSVD. This association remained robust even after adjusting for competitive events, underscoring its stability. In previous investigations, FAR has demonstrated notable associations with prognosis in various medical conditions. Studies have linked FAR to prognostic indicators in different cancers, severity in obstructive sleep apnea, acute coronary artery disease, development of cardiac autonomic neuropathy in diabetic patients, and ischemic retinal vein occlusion12,14,23–26 In the realm of cerebrovascular disease, Ruan Y et al conducted a study involving 256 consecutive stroke patients with comorbid hypertension (HT) and 256 age- and sex-matched non-HT stroke patients. Their analysis revealed that a high FAR was independently associated with an increased risk of hemorrhagic transformation (HT) after acute ischemic stroke (AIS).27 Similarly, leveraging data from the third Chinese Stroke Registry (CNIS-III) survey, Wang X et al found that elevated FAR (>11.44) heightened the risk of short- and long-term poor functional Outcomes, encompassing disability and all-cause mortality, in patients with AIS.28 Furthermore, Wang X et al revealed that FAR ≥0.077 on admission may be an independent predictor of death/disability at 3 months after lacunar stroke by analyzing 393 patients with acute lacunar stroke.29 These findings collectively underscore the utility of FAR in both small and large cerebrovascular diseases, providing valuable insights into its prognostic significance across diverse cerebrovascular conditions.
There is a compelling correlation between vascular inflammatory markers (eg, adhesion molecules, von Willebrand factor [VWF]) and CSVD.11 Elevated chronic inflammatory markers are posited as essential for the risk and progression of CSVD, influencing vascular endothelial cell damage, neurovascular unit alterations, and white matter integrity decline. Endothelial cell destruction-induced dysfunction can lead to BBB breakdown, increasing permeability and allowing immune cells entry into the brain. This cascade contributes to changes in small blood vessel structure, thin-walled tissues, and perivascular spaces, collectively fostering CSVD development.10,30 Several studies emphasize endothelial cell destruction and BBB leakage as initial pathologic hallmarks, suggesting inflammation as a potential driver of CSVD.31,32 Consequently, focusing on inflammatory marker changes becomes crucial for early CSVD risk identification. Importantly, a notable study indicated an overall shift in blood protein patterns, characterized by increased fibrinogen and globulin levels and decreased albumin concentrations, as associated with subsequent vascular events (stroke, MI, or vascular death).33 This highlights the potential value of FAR in predicting IS and CVE.
Fibrinogen and ALB, both synthesized by the liver, play distinct roles in the context of stroke and cardiovascular events. Fibrinogen is intricately linked to platelet aggregation, fibrin formation, and heightened plasma viscosity, serving as a marker for systemic inflammation.34,35 Its involvement extends to disrupting endothelial cell integrity and increasing BBB permeability into the central nervous system, potentially leading to neuronal damage. This, in turn, triggers immune cell recruitment and the release of pro-inflammatory factors, culminating in a neuroinflammatory response.36–38 Resch et al found that hyperfibrinogenemia was an independent risk factor for cardiovascular events in stroke survivors by following up 625 first-time stroke patients (mean time 2 years).39 Previous studies have shown that high levels of fibrinogen increase the risk of IS.40 Conversely, ALB exhibits neuroprotective properties by reducing BBB permeability and engaging in anti-apoptotic, antioxidant, and anti-inflammatory processes. The enduring endothelium-protective and antioxidant effects of ALB contribute significantly to recovery from cerebral ischemia. Furthermore, ALB may inhibit fibrinogen activity, thus reducing fibrin accumulation and participating in processes such as the inhibition of platelet function and thrombosis.41–46 In addition, ALB levels reflect the nutritional status of the body, and a low ALB level may indicate that the individual is malnourished with a higher likelihood of vascular events in the future, increasing the risk of stroke recurrence. The Results of a meta-analysis of 14 studies showed that a low plasma ALB level was independently associated with an increased risk of developing cardiovascular disease events.47 In a study following 753 consecutive patients with acute ischemic stroke for one year, Zhang et al found that lower serum ALB levels were associated with an elevated risk of recurrence.48 An intricate interplay was observed between fibrinogen and ALB, substantiating the role of FAR as a reflective measure of the body’s inflammatory response.
Our study further elucidated a linear relationship between FAR and IS, as well as the incidence of CVE in patients with CSVD. The optimal cutoff value of 8.69 was identified through calculations, providing a practical benchmark for clinicians. In addition, the results of our subgroup analyses also revealed some specific populations in which IS and CVE occurred at high FAR levels, Based on the analysis of the robustness of the results, we concluded that high FAR levels could significantly affect IS and CVE in individuals aged more than 60 years. Studies showed that the plasma fibrinogen concentration increased with age, whereas there were no age-related changes in albumin synthesis rate and concentration.49,50 This seemed to indicate that FAR levels may be higher in the body at a higher age, and this chronic inflammatory state was more likely to contribute to the development of vascular events.
Our study has a few limitations. Firstly, we only examined the relationship between baseline FAR and IS/CVE risk, neglecting trend changes in FAR, warranting further investigation. Secondly, as a single-center study, expanding the sample size and validating results in a multicenter population is crucial for broader applicability. Thirdly, our study was conducted exclusively in a Chinese population, necessitating validation in diverse populations to assess generalizability.
Conclusion
In Conclusion, our study revealed a significant and linear association between elevated FAR and the risk of IS, as well as the development of CVE in CSVD patients. Our findings suggested that as FAR surpassed 8.69, the risk escalated proportionally, with a particularly noteworthy impact observed among individuals aged over 60 years.
Data Sharing Statement
Data are available upon reasonable request from the corresponding author (E-mail address: [email protected]).
Ethical Approval and Informed Consent
Our study was approved by the Ethics Committee of the first Affiliated Hospital of Zhengzhou University (Ethics Review Number: 2021-KY-1059-002). All procedures carried out in studies involving human participants are consistent with the ethical standards of institutions and/or national research councils, as well as with the 1964 Helsinki Declaration and its subsequent amendments or similar ethical standards. All patients signed an Informed consent.
Acknowledgments
We thank all the participants of the present study.
Author Contributions
All authors made a significant contribution to the work reported, whether that is in the conception, study design, execution, acquisition of data, analysis and interpretation, or in all these areas; took part in drafting, revising or critically reviewing the article; gave final approval of the version to be published; have agreed on the journal to which the article has been submitted; and agree to be accountable for all aspects of the work.
Funding
This work was funded by The Stroke Prevention and Treatment Technology Research project (WKZX2023CZ0302).
Disclosure
The authors declare no conflicts of interest related to this study.
References
1. Chen X, Wang J, Shan Y, et al. Cerebral small vessel disease: neuroimaging markers and clinical implication. J Neurol. 2019;266(10):2347–2362. doi:10.1007/s00415-018-9077-3
2. Duering M, Biessels GJ, Brodtmann A, et al. Neuroimaging standards for research into small vessel disease—advances since 2013. Lancet Neurol. 2023;22(7):602–618. doi:10.1016/s1474-4422(23)00131-x
3. Mehta T, McClure LA, White CL, Taylor A, Benavente OR, Lakshminarayan K. Effect of postural hypotension on recurrent stroke: secondary prevention of small subcortical strokes (SPS3) Study. J Stroke Cerebrovasc Dis. 2019;28(8):2124–2131. doi:10.1016/j.jstrokecerebrovasdis.2019.04.009
4. Hoshino H, Toyoda K, Omae K, et al. Dual antiplatelet therapy using cilostazol with aspirin or clopidogrel: subanalysis of the CSPS.com trial. Stroke. 2021;52(11):3430–3439. doi:10.1161/strokeaha.121.034378
5. Ling Y, De Guio F, Duering M, et al. Predictors and clinical impact of incident lacunes in cerebral autosomal dominant arteriopathy with subcortical infarcts and leukoencephalopathy. Stroke. 2017;48(2):283–289. doi:10.1161/strokeaha.116.015750
6. Chen CH, Cheng YW, Chen YF, Tang SC, Jeng JS. Plasma neurofilament light chain and glial fibrillary acidic protein predict stroke in CADASIL. J Neuroinflammation. 2020;17(1):124. doi:10.1186/s12974-020-01813-5
7. Bamford J, Sandercock P, Jones L, Warlow C. The natural history of lacunar infarction: the Oxfordshire community stroke project. Stroke. 1987;18(3):545–551. doi:10.1161/01.str.18.3.545
8. Soda T, Nakayasu H, Maeda M, et al. Stroke recurrence within the first year following cerebral infarction–Tottori University Lacunar Infarction Prognosis Study (TULIPS). Acta Neurol Scand. 2004;110(6):343–349. doi:10.1111/j.1600-0404.2004.00290.x
9. Arboix A, Font A, Garro C, García-Eroles L, Comes E, Massons J. Recurrent lacunar infarction following a previous lacunar stroke: a clinical study of 122 patients. J Neurol Neurosurg Psychiatry. 2007;78(12):1392–1394. doi:10.1136/jnnp.2007.119776
10. Low A, Mak E, Rowe JB, Markus HS, O’Brien JT. Inflammation and cerebral small vessel disease: a systematic review. Ageing Res Rev. 2019;53:100916. doi:10.1016/j.arr.2019.100916
11. Rouhl RP, Damoiseaux JG, Lodder J, et al. Vascular inflammation in cerebral small vessel disease. Neurobiol Aging. 2012;33(8):1800–1806. doi:10.1016/j.neurobiolaging.2011.04.008
12. Sun H, Ma J, Lu J, et al. Fibrinogen-to-albumin ratio predicts overall survival of hepatocellular carcinoma. World J Gastrointest Oncol. 2023;15(9):1662–1672. doi:10.4251/wjgo.v15.i9.1662
13. Yang M, Tang L, Bing S, Tang X. Association between fibrinogen-to-albumin ratio and hemorrhagic transformation after intravenous thrombolysis in ischemic stroke patients. Neurol Sci. 2023;44(4):1281–1288. doi:10.1007/s10072-022-06544-4
14. Hwang KT, Chung JK, Roh EY, et al. Prognostic influence of preoperative fibrinogen to albumin ratio for breast cancer. J Breast Cancer. 2017;20(3):254–263. doi:10.4048/jbc.2017.20.3.254
15. Wang Y, Bai L, Li X, Yi F, Hou H. Fibrinogen-to-albumin ratio and clinical outcomes in patients with large artery atherosclerosis stroke. J Am Heart Assoc. 2023;e030837. doi:10.1161/jaha.123.030837
16. Wardlaw JM, Smith EE, Biessels GJ, et al. Neuroimaging standards for research into small vessel disease and its contribution to ageing and neurodegeneration. Lancet Neurol. 2013;12(8):822–838. doi:10.1016/s1474-4422(13)70124-8
17. Dong G, Ma T, Xu Z, et al. Fibrinogen-to-albumin ratio in neonatal sepsis. Int J Gen Med. 2023;16:4965–4972. doi:10.2147/ijgm.S432903
18. Xu Z, Li F, Wang B, et al. New insights in addressing cerebral small vessel disease: association with the deep medullary veins. Front Aging Neurosci. 2020;12:597799. doi:10.3389/fnagi.2020.597799
19. Chen X, Wei L, Wang J, et al. Decreased visible deep medullary veins is a novel imaging marker for cerebral small vessel disease. Neurol Sci. 2020;41(6):1497–1506. doi:10.1007/s10072-019-04203-9
20. Chang JY, Lee JS, Kim BJ, et al. Influence of hemoglobin concentration on stroke recurrence and composite vascular events. Stroke. 2020;51(4):1309–1312. doi:10.1161/strokeaha.119.028058
21. van Wijk I, Kappelle LJ, van Gijn J, et al. Long-term survival and vascular event risk after transient ischaemic attack or minor ischaemic stroke: a cohort study. Lancet. 2005;365(9477):2098–2104. doi:10.1016/s0140-6736(05)66734-7
22. Misquitta K, Daou M, Conklin J, et al. Detecting silent acute microinfarcts in cerebral small vessel disease using submillimeter diffusion-weighted magnetic resonance imaging: preliminary results. Stroke. 2022;53(7):e251–e252. doi:10.1161/strokeaha.122.039723
23. Makkar K, Sharma YP, Batta A, Hatwal J, Panda PK. Role of fibrinogen, albumin and fibrinogen to albumin ratio in determining angiographic severity and outcomes in acute coronary syndrome. World J Cardiol. 2023;15(1):13–22. doi:10.4330/wjc.v15.i1.13
24. Hizli O, Cayir S, Coluk Y, Kayabasi S, Yildirim G. The novel indicators of moderate to severe sleep apnea: fibrinogen to albumin ratio vs. CRP to albumin ratio. Eur Arch Otorhinolaryngol. 2021;278(3):851–855. doi:10.1007/s00405-019-05770-5
25. Zhao S, Yang Z, Yu M, et al. Influence of fibrinogen/albumin ratio and fibrinogen/pre-albumin ratio on cardiac autonomic neuropathy in type 2 diabetes. Diabetes Metab Syndr Obes. 2023;16:3249–3259. doi:10.2147/dmso.S431551
26. Guclu H, Ozal SA, Pelitli Gurlu V, Özgün GS, Özgün E. Increased fibrinogen to albumin ratio in ischemic retinal vein occlusions. Eur J Ophthalmol. 2017;27(6):735–739. doi:10.5301/ejo.5000942
27. Ruan Y, Yuan C, Liu Y, et al. High fibrinogen-to-albumin ratio is associated with hemorrhagic transformation in acute ischemic stroke patients. Brain Behav. 2021;11(1):e01855. doi:10.1002/brb3.1855
28. Wang X, Pan Y, Zhang R, et al. Association between fibrinogen-to-albumin ratio and adverse stroke outcomes among patients with acute ischemic stroke. Cerebrovasc Dis. 2023:1–9. doi:10.1159/000535303
29. Zheng L, Wang Z, Liu J, et al. Association between admission blood fibrinogen-to-albumin ratio and clinical outcomes after acute lacunar stroke. Biomarker Med. 2021;15(2):87–96. doi:10.2217/bmm-2019-0537
30. Evans LE, Taylor JL, Smith CJ, Pritchard HAT, Greenstein AS, Allan SM. Cardiovascular comorbidities, inflammation, and cerebral small vessel disease. Cardiovasc Res. 2021;117(13):2575–2588. doi:10.1093/cvr/cvab284
31. Bailey EL, Wardlaw JM, Graham D, Dominiczak AF, Sudlow CL, Smith C. Cerebral small vessel endothelial structural changes predate hypertension in stroke-prone spontaneously hypertensive rats: a blinded, controlled immunohistochemical study of 5- to 21-week-old rats. Neuropathol Appl Neurobiol. 2011;37(7):711–726. doi:10.1111/j.1365-2990.2011.01170.x
32. Rajani RM, Quick S, Ruigrok SR, et al. Reversal of endothelial dysfunction reduces white matter vulnerability in cerebral small vessel disease in rats. Sci Transl Med. 2018;10(448). doi:10.1126/scitranslmed.aam9507
33. Beamer N, Coull BM, Sexton G, de Garmo P, Knox R, Seaman G. Fibrinogen and the albumin-globulin ratio in recurrent stroke. Stroke. 1993;24(8):1133–1139. doi:10.1161/01.str.24.8.1133
34. Davalos D, Akassoglou K. Fibrinogen as a key regulator of inflammation in disease. Semin Immunopathol. 2012;34(1):43–62. doi:10.1007/s00281-011-0290-8
35. Machlus KR, Cardenas JC, Church FC, Wolberg AS. Causal relationship between hyperfibrinogenemia, thrombosis, and resistance to thrombolysis in mice. Blood. 2011;117(18):4953–4963. doi:10.1182/blood-2010-11-316885
36. Petersen MA, Ryu JK, Akassoglou K. Fibrinogen in neurological diseases: mechanisms, imaging and therapeutics. Nat Rev Neurosci. 2018;19(5):283–301. doi:10.1038/nrn.2018.13
37. Lominadze D, Dean WL, Tyagi SC, Roberts AM. Mechanisms of fibrinogen-induced microvascular dysfunction during cardiovascular disease. Acta Physiol (Oxf). 2010;198(1):1–13. doi:10.1111/j.1748-1716.2009.02037.x
38. Merlini M, Rafalski VA, Rios Coronado PE, et al. Fibrinogen induces microglia-mediated spine elimination and cognitive impairment in an alzheimer’s disease model. Neuron. 2019;101(6):1099–1108.e6. doi:10.1016/j.neuron.2019.01.014
39. Resch KL, Ernst E, Matrai A, Paulsen HF. Fibrinogen and viscosity as risk factors for subsequent cardiovascular events in stroke survivors. Ann Intern Med. 1992;117(5):371–375. doi:10.7326/0003-4819-117-5-371
40. Hou HQ, Xiang XL, Pan YS, et al. Baseline or 90-day fibrinogen levels and long-term outcomes after ischemic stroke or TIA: results from the China national stroke registry III. Atherosclerosis. 2021;337:35–41. doi:10.1016/j.atherosclerosis.2021.10.002
41. Oettl K, Stauber RE. Physiological and pathological changes in the redox state of human serum albumin critically influence its binding properties. Br J Pharmacol. 2007;151(5):580–590. doi:10.1038/sj.bjp.0707251
42. Margarson MP, Soni N. Serum albumin: touchstone or totem? Anaesthesia. 1998;53(8):789–803. doi:10.1046/j.1365-2044.1998.00438.x
43. Gresele P, Deckmyn H, Huybrechts E, Vermylen J. Serum albumin enhances the impairment of platelet aggregation with thromboxane synthase inhibition by increasing the formation of prostaglandin D2. Biochem Pharmacol. 1984;33(13):2083–2088. doi:10.1016/0006-2952(84)90577-x
44. Belayev L, Liu Y, Zhao W, Busto R, Ginsberg MD. Human albumin therapy of acute ischemic stroke: marked neuroprotective efficacy at moderate doses and with a broad therapeutic window. Stroke. 2001;32(2):553–560. doi:10.1161/01.str.32.2.553
45. Prajapati KD, Sharma SS, Roy N. Current perspectives on potential role of albumin in neuroprotection. Rev Neurosci. 2011;22(3):355–363. doi:10.1515/rns.2011.028
46. Galanakis DK. Anticoagulant albumin fragments that bind to fibrinogen/fibrin: possible implications. Semin Thromb Hemost. 1992;18(1):44–52. doi:10.1055/s-2007-1002409
47. Ronit A, Kirkegaard-Klitbo DM, Dohlmann TL, et al. Plasma albumin and incident cardiovascular disease: results from the CGPS and an updated meta-analysis. Arterioscler Thromb Vasc Biol. 2020;40(2):473–482. doi:10.1161/atvbaha.119.313681
48. Zhang Q, Lei YX, Wang Q, et al. Serum albumin level is associated with the recurrence of acute ischemic stroke. Am J Emerg Med. 2016;34(9):1812–1816. doi:10.1016/j.ajem.2016.06.049
49. Hager K, Seefried G, Felicetti M, Platt D. Plasma fibrinogen – are there age-dependent changes? Arch Gerontol Geriatr. 1994;19 Suppl 1:99–106. doi:10.1016/s0167-4943(05)80054-8
50. Fu A, Nair KS. Age effect on fibrinogen and albumin synthesis in humans. Am J Physiol. 1998;275(6):E1023–30. doi:10.1152/ajpendo.1998.275.6.E1023