Tech
Spermbots and their applications in assisted reproduction | IJN
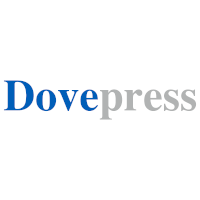
Introduction
According to the latest WHO report, infertility affects about 1 in 6 reproductive-aged couples, which has been a serious health and social burden.1 Male factors are estimated to be responsible for 50% of infertility cases.2 The causes of male infertility or subfertility are complex, including but not limited to genetic abnormalities, anatomical defects, erectile dysfunction, hormonal disorders, oxidative stress, and psychoactive drugs.3 However, it is known that up to 90% of male problems will end up to low sperm count or poor sperm quality. Thus, semen analysis is the most widely used as well as the most efficient laboratory testing for the diagnosis of male infertility. The reference standard values for the main semen parameters tend to be lower in each new edition of the WHO manual for human semen analysis.4 For example, the lower limit value of semen volume has changed from 2 mL to 1.4 mL, and the lower limit percentage of progressive sperm motility has decreased from 60% to 30%. On the other hand, the recent retrospective study and meta-analysis showed that human sperm quality is decreasing dramatically during the past decades, due to the issues of environmental exposure and unhealthy lifestyle.5,6
The assisted reproductive technologies (ART) offer ultimate solutions for the treatment of infertility. There are already many options for male factor infertility in clinical practices.7 Generally, the cases of sperm-related infertility can be classified into two main categories: with or without mature sperms in the seminal fluid. For the first condition, the in vitro fertilization (IVF) is usually used for oligospermia patients with low sperm count. While the intracytoplasmic sperm injection (ICSI), which directly injects a single sperm into the oocyte, is effective for asthenospermia patients with low sperm motility. If mature sperms cannot be detected in the semen, surgical approaches via epididymal or testicular sperm extraction can be used to obtain sperm cells for obstructive azoospermia patients. Finally, for the cases of non-obstructive azoospermia or testicular cancer, donor sperms from the sperm bank can be used for the subsequent procedure of intrauterine insemination (IUI). Although ART has successfully helped the birth of millions of infants to date, a large portion of cycles still fail.8 Besides, ART procedures are usually time-consuming and expensive.9 Hence, it is still necessary to develop novel technologies in this field to further improve the success rate, reduce the cost, and shorten the cycle time.
As early as in 2013, the first sperm-based biohybrid micro-robot was developed combing a motile sperm cell with a rolled-up magnetic microtube, which can be remotely controlled by an external magnetic field.10 The so-called “spermbot” opens a new window for us to develop fascinating applications in the area of ART. Over the past decade, there has been considerable progress in the designing and optimizing of various spermbots or sperm-like nanorobots. There are two main directions for the potential applications of spermbots: infertility treatment and drug delivery. However, the translation of these nanorobots into clinical practice is lagging behind, especially in the field of ART. Most of the researchers of spermbots are only experts in nanomaterials or nanoengineering. Previous design considerations of spermbots may not properly meet the actual clinical needs to serve ART. Moreover, the safety of nanomaterials may be the major barrier to bring hybrid spermbots from bench to bedside.11 Hence, multidisciplinary discussion and collaboration are needed to refine the design of spermbots and to accelerate the translation.
In recent years, several broad reviews have already been published to summarize the design, control mechanism and potential applications of all types of biohybrid microrobots or sperm-driven microrobots.12,13 However, as mentioned above, these reviews mostly represent the viewpoints of nanoengineering researchers. The discussion of the applications is also mainly focused on targeted drug delivery. Thus, it is urgent and necessary to conduct an updated review and perspective from the viewpoints of the researchers and clinicians in the field of reproductive medicine. In the present review, we will first update and summarize the structural design and functional control of spermbots. Then, focusing on the area of ART, we will draw up a comprehensive and constructive blueprint for the applications of spermbots, considering the corresponding sperm related clinical issues in the real-world. Finally, we will also propose a roadmap to overcome the challenges and barriers. We believe this perspective will help us to refine the design of spermbots, as well as to promote the translation of spermbots in clinical practice.
The Design and Control of Spermbots
The Structural Design and Classification of Spermbots
Consistent with previous publications,12,14 the “spermbot” is defined as sperm cell driven biohybrid microrobot. The structure of a mature mammalian sperm can be divided into three distinct parts: head, midpiece and tail.15 Specifically, the head region contains haploid chromosomes, which are the paternal hereditary materials for the formation of a zygote (Figure 1A). The midpiece region is formed by the tightly wrapping of mitochondrial sheath around the axoneme. Thus, the midpiece is thought to produce the energy to fuel sperm motility. Finally, the tail or flagella region is a specialized form of the cilium, which is the basic component for the driving force of sperm movement.
The hybrid design of a spermbot is the combination of a biological sperm cell with specific nano decorations. To date, five basic types of nano-components have been developed for the production of spermbots, including microtube, microhelice, tetrapod microtube, rice grain-shaped (or spindle-type) nanoparticle and the complex nanocarrier (Figure 1B). The first three types of nano components are used for the fabrication of one-to-one paired spermbots. The conical microtube is fabricated by rolling up functional nanomembranes on photoresist and can trap sperm head into its hollow cavity.10 Due to the emerging and development of nanoscale three-dimensional (3D) printing techniques, it is also able to directly construct complex nano components. For example, the microhelical polymer can be produced by 3D direct laser writing (also known as 3D lithography) and can be coupled to the midpiece or tail region of a sperm.16 The dedicatedly designed tetrapod (with four flexible arched arms in a microtube) can be directly printed by two-photon 3D nanolithography and be coupled to a sperm head.17 In addition to these one-to-one nano widgets, one single sperm cell can also be coupled with multiple nanoparticles. For example, the head or tail part of a sperm can be coated with rice grain-shaped nanoparticles (fabricated by chemical aging) via electrostatic self-assembly, which is known as the IRONSperm.18 Compared to those one-to-one targeted widgets, the self-assembly process of many-to-one nanoparticles is more efficient than the capturing or trapping process with highly randomness. Moreover, a recent study also developed a many-to-many sperm cluster based on the IRONSperms via magnetically assembly, which enables wireless rolling locomotion and noninvasive localization simultaneously.19 Finally, a recently designed complex magnetic microcarrier, which can also be fabricated by two-photon lithography, is capable of carrying multiple sperms.20 This type of one-to-many nano components could be used to control the dose of delivered cargos (such as targeted drugs).
Besides the above-mentioned biohybrid spermbot, there are many types of sperm mimics, which are also helpful for us to understand the regulation of sperm motility, as well as to develop functional nanobots. Here, we define these sperm mimics as sperm-like nanobots, and classify them into two subcatalogs: flagellate microorganisms and biomimetic sperms (Figure 1C). First, inspired by the feature of self-propelled swimming, several types of flagellated bacteria (such as salmonella typhimurium or Escherichia coli) can act as the motor part of a hybrid microrobot for targeting therapy. The flagellated bacteria can be attached to the targeted nanoparticles via antibody or hydrophobic binding.21,22 Similar to spermbots, the motility of bacteriobot can also be enhanced by the decoration of magnetic particles.23 Moreover, compared to the canonical flagellated bacteria, the flagellated magnetotactic bacteria (such as MO-1) contains self-produced magnetosome chain with magnetoresponsive capability.24 The dual functions of magnetotactic bacteria make it become a hotspot to develop microrobots for cell screening, drug delivery and disease detection.25,26 Second, several types of artificial sperms have been developed to mimic sperm movement. The simplest structure of a biomimetic sperm is fabricated by combining a head (iron-oxide nanoparticle) and a tail (polystyrene).27 The length and swimming speed of this soft microrobotic sperm are over 300 μm and 100 μm/s, respectively. A recent study also produced a flexible sperm-like nanorobot, by the self-assembling of a superparamagnetic head (biotin-coated Fe3O4 nanoparticles) and a flexible flagellum (a nanowire with the Au end modified with streptavidin).28 This type of sperm-like nanorobot is relatively smaller with a length of 15 μm, and swims at a speed of 3–4 μm/s. The average length and velocity of human sperm are approximately 50 μm and 80 μm/s, respectively.29,30 Although these sperm-like nanowires do not fully mimic the size and movement of real sperm cells, they provide valuable insights for the design and control of spermbots. Finally, a recent simulation study proposed a novel design of autonomous sperm based on piezoelectric technique, which aims to fully emulate sperm structure and functionalities.31 This conceptual design of a cyborg sperm is difficult to be implemented in the near future because of the size limitation of the microcontroller and battery, which cannot be produced at the nanoscale. However, piezoelectric nanowires have been fabricated and can be controlled by the magnetic field for drug delivery.32
Currently, the designed applications of spermbot are also fast-evolving and can be divided into three stages (Figure 1D). First, the early studies only focused on the remote control of sperm movement.10,16 Then, the following studies proved the drug loading abilities of spermbots.17–20 At the meantime, the biocompatibility and treatment effect are also confirmed in 3D ovarian cancer cells. The recent study successfully constructed the multifunctional spermbot based on 4D printed microcarrier.33 This revolutionary microcarrier can not only deliver multiple sperms, but can also control sperm capacitation and sperm-dependent degradation of cumulus cells.
The Control and Functionality Design of Spermbots
The initial motivation for designing hybrid spermbots is to remotely control the direction of motile sperms. For those sperms with motion deficiencies, nano components can also be served as micromotors to indirectly transport sperm cells. Hence, almost all types of nano components for spermbots are magnetically or paramagnetically processed, which enables the control of the movements of spermbots by using an external magnetic field (Figure 2A). The magnetical nanomaterials also make it possible to monitor the real-time localization of spermbots in vivo via various imaging methods such as magnetic resonance imaging and X-ray computed tomography.34 Another remote technology that could be used for both driving the movement and monitoring the localization of nanorobots is the ultrasound.35,36 Moreover, the remote control can also be used to help assemble and disassemble hybrid spermbots. For example, the magnetic microhelix can be removed from the sperm cell by inverting the original magnetic field.16 Another method to remotely control the release of sperm cells from spermbots is by using external temperature stimulus. For example, when the temperature increases to 38 °C, the thermoresponsive microtubes are unfolding to release the captured sperm cells.37 In addition to the dynamic control of nano components, the cell part of spermbots could also be regulated via chemical stimulation. For example, to ensure that the sperm cells can escape from the bended tetrapod, progesterone is added into the medium to obtain sperms with hyperactivated motility.17 Additionally, in a recently developed multifunctional sperm microcarrier, temperature change is used to control the release of heparin for sperm hyperactivation, and pH change is used to control the action of hyaluronidase for the removal of cumulus cells.33 Finally, the above mentioned sperm-like soft microbots also offer instructions and inspirations for the design of novel types of hybrid spermbots that can be controlled and actuated by chemical, electric or light.38–40
To obtain an overview of the functionality design and control of spermbot, we comprehensively summarized the functional features of a biological sperm. These features can be classified into four main categories: motility, movement tendency, competition/cooperation, and fertilization (Figure 2B). First, it is well known that sperms are swimming cells based on the self-propelled flagella. However, sperm motility can further be divided into four different grades, including immotile (unable to move), non-progressive movement (slightly moving but not progressing forward), progressive movement (swimming linearly or in a large circle), and hyperactivated motility (flagella beat asymmetrically with increased bend amplitude).41 Second, the moving direction of sperm cells can be guided by many attractive factors, which can be classified into two main classes (the active and the passive processes). The active processes contain two phenomena: chemotaxis (attracted by a higher concentration of chemical, such as progesterone)42 and thermotaxis (attracted by a relative higher temperature, from 31 to 37°C).43 A previous study showed that chemotaxis is the basic mechanism for different females to consistently and differentially selects sperms from specific males.44 The passive processes include rheotaxis (swimming against fluid flows)45 and thigmotaxis (swimming along surfaces).46 Third, sperm competition is a common phenomenon for many species to shape sperm phenotypes (sperm number, sperm size and egg–sperm interactions) evolutionary.47 A recent study based on genetic mouse models showed that some sperms may win the competition by poisoning the surrounding competitors.48 On the other hands, competition between different individuals or species may also drive the actions of sperm cooperation. For example, it was found that the sperms of deer mice tend to aggregate more often within conspecific sperms than heterospecific sperms, based on the apical hook structure in the head.49 Although human sperm lacks such a hook structure, it has also been reported that human sperms can be clustered into groups by hydrodynamic force in viscoelastic fluids.50 Finally, the ultimate goal of a sperm is to fertilize an oocyte. The key functional steps of sperm–egg interaction includes: sperm binds to the zona pellucida via the recognition of surface receptors, sperm digests the zona pellucida by releasing the enzymes stored in the acrosome, and sperm fuses to the egg plasma membrane via the surface proteins located at the side of sperm head.51 Conclusively, the above mentioned functions provided a full reference list to reconsider the design and control of spermbots. Some of the features, such as sperm motility and chemotaxis, have been adopted in the current design of spermbots. However, an integrated reviewing of these characteristics could provide new directions to improve the automated selection, clustering delivery, targeted binding and enzymatic digestion of hybrid spermbots.
The Blueprint and Roadmap for Translating Spermbots into Clinical Practice
The Blueprint for the Applications of Spermbots
The potential applications of all types of nanobots are broad, including but not limited to disease diagnosis, assisted surgeries, drug delivery, target therapeutics, and biological imaging.52,53 Although spermbot can also be applied in many fields like other types of nanobots, the cell part of spermbot determines its fundamental and promising applications in the field of assisted reproduction. To maximally explore the potential applications of spermbots, we comprehensively summarized the journey of human sperms (from their production to fertilization), and the corresponding dysfunctions associated with infertility (Figure 3). First, the sperm cells are periodically produced in the seminiferous tubules of testis via the complex process of spermatogenesis, which involves sequential division and differentiation of spermatogonial stem cells, spermatocytes, round spermatids and elongated sperms.54 Then, testicular sperms acquire the forward motility during the maturation process in the epididymis.55 When the sperms are ejaculated into the female reproductive tract, they need to travel through the vagina, cervix and uterus to reach the fallopian tube.56 During this challenging journey, only a small portion of sperms can pass the natural selection environments, such as the acidic vaginal fluid, the immunological defenses, prematurely capacitating factors and the narrowness of the uterotubal junction (the isthmus). The remaining sperms are deposited in the ampulla of the fallopian tube and can only survive for up to a few days. Finally, only capacitated sperms with acrosomal responsiveness and hyperactivity are capable of penetrating the cumulus cells, digesting the zona pellucida and fertilizing an oocyte.
Correspondingly, sperm-related abnormal conditions may occur at any stage and lead to male infertility or subfertility. First, nonobstructive azoospermia is the most serious condition that cannot produce any mature sperm cells.57 Another serious condition of completely losing fertility is the testicular cancer, which is usually occurring in young males.58 The currently developed technologies are able to generate in vitro differentiated sperm cells from stem cells or spermatocytes, offering potential solutions to restore male fertility.59,60 More importantly, the recent developed genome editing technologies based on CRISPR-Cas9 also make it possible to correct genetic diseases in the germ cells.61,62 However, these regenerated “sperm cells” are actually presumptive spermatids or sperm-like cells without specialized head and flagellum structures. Thus, these immature sperms could be equipped with artificial micromotors to help their movements. In addition, these cells are also lack of function to digest the zona pellucida. Hence, the design of the corresponding nanocomponents should also be able to implement the function of acrosome, which could be achieved by the stimuli-responsible microcarrier.33 Second, as mentioned in the above, human sperm quality is dramatically declining over the past decades and results in the increase of oligospermia (low sperm count), asthenospermia (low sperm motility), and teratozoospermia (abnormal sperm morphology).63 It is obvious that the current design of spermbots mainly aims to solve the problem of low sperm motility by the hybrid of nano propeller. However, the microcarrier or self-assembled nanoparticles could also be used to enrich and transport sperm clusters to improve the possibility of fertilization. Additionally, the complex environments of the female reproductive tract may also reduce the survival and motility of sperms, due to inflammation, oxidative damage and antisperm antibodies.64–66 Thus, the nanocomponent parts of spermbots can be coupled with drugs and designed to be responsively released to treat these conditions. Besides the immunological barriers, female reproductive tract may also preferentially select sperms from specific males based on chemotaxis.44 Hence, the designs of anti-immune or impartially selected spermbots are fascinating new directions in the future, which could be implemented by combining drug-loaded nanocomponents or coupling into multifunctional microcarrier. Finally, as a biocompatible transporter, spermbot can also be used for targeted drug delivery to treat gynecologic diseases (such as infection and inflammation) and tumors (such as cervical cancer, endometrial cancer or ovarian cancer) in the female genital tract. For example, a sperm-based drug-delivery system has been established and was shown to successfully treat patient-representative ovarian cancer cell cultures in vitro.20
The Roadmap for Translating Spermbots into Clinical Practice
The technologies of fabricating various spermbots are fast evolving, mainly due to the emerging of nanoscale three-dimensional (3D) printing platforms.67 However, as mentioned above (Figure 1D), the applicational development of spermbots is still staying at a preliminary stage (Figure 4A). All of the functional studies are based on in vitro experiments. Most of the germ cells (including sperms, cumulus and oocytes) are from bovine origin. Only one study fabricated human spermbots and verified their therapeutic effects on patient-representative 3D ovarian cancer cells.20 Although these studies have shown that spermbots have promising applications in the field of assisted reproduction, there is still a long way to go before they are ready for clinical usage. For the translating of new ARTs, the European Society of Human Reproduction and Embryology (ESHRE) has raised a four-step research chain: animal studies, preclinical embryo research, clinical trials and follow-up studies.68 Thus, the future studies of spermbots are also recommended to follow this guidance.
Before going on to the stage of clinical trials, there are many challenges and risks to be solved for translating the design of spermbots into clinical practice. First, the nano components of spermbots contain complex nanomaterials that may cause the problems of biocompatibility. Some of the designs are capable of controlled removal of the nano components (such as the magnetic microhelix) via the magnetic force.16 However, this process only disassembles the spermbots but not completely clear the nano components, which may hinder the in vivo applications of spermbots. Hence, the development of biodegradable nano components could offer potential solutions to provide a contamination-free environment.69 Besides, the possible existence of bacteria contaminations and anti-sperm antibodies should also be noticed and solved before preparing sperm cells to avoid infection or immune response.70,71 Second, it is relatively easy to build in vitro controlling and imaging system for spermbots. However, the safety and effectiveness of remote control and image monitor should be evaluated and validated before translating into in vivo applications. Finally, it well known that the use of assisted reproductive technologies has been accompanied by a wide range of complex ethical and legal problems.72 For example, it is still debating whether to fully embrace the genome edited germ cells in human reproduction.73 Spermbots also face similar problems, as they may skip the steps of natural selection in the female reproductive tract or be combined with the genome edited sperm cells. Thus, even when the technologies of spermbots are ready and applicable for clinical practice, it cannot be overemphasized that ethical and legal issues should always be considered before technical promotion.
Considering the above issues, we thus proposed the “three laws” for the clinical applications of spermbots in assisted reproduction: good genetics, gentle operation and no contamination. First, although spermbots can be used to drive all types of abnormal sperms technically, it should be noted that only sperms with good genetics can be used for fertilizing an oocyte. Many cases of poor sperm quality are actually caused by various factors such as unhealthy lifestyle and inflammation.7 Most of them can and should be cured to obtain normal sperm cells. Then, spermbots based solutions can be considered for other complex cases of idiopathic male infertility or azoospermia. Before the production of spermbots, we suggest to perform genetic diagnosis for the sperm donors to avoid adverse outcomes and hereditary diseases. Semen analysis must be performed to double-check the DNA integrity and morphology of sperm cells. The above processes can also be replaced by directly using the technology of preimplantation genetic diagnosis after fertilization. However, this law is not applicable to therapeutical spermbots. Second, the whole procedure of handling spermbots should be noninvasive or minimally invasive. This requires the controlling and imaging platforms to be more comfortable with the human body. Finally, since fertilization is only the beginning of a new life, more caution is needed for assisted reproduction to avoid long-term effects on the embryo. The nano components of spermbots should be completely removed or degraded to offer a safe environment for the subsequent embryo development. For the treatment of reproductive tract diseases, it is also important to avoid introducing additional nano contaminants.
To prospectively evaluate the application values of spermbots, we further compare the procedures of spermbots based technologies with the traditional assisted reproductive technologies for treating male subfertility (Figure 4B), including intrauterine insemination (IUI), in vitro fertilization (IVF), and intracytoplasmic sperm injection (ICSI). Specifically, the IUI is performed by directly injecting the sperm cells into the uterus of female recipient, which is applicable for poor sperm quality or survival.74 The IVF is achieved by the mixture of the sperms and the targeted oocyte in a culture dish. It is mainly prepared for the following embryo development and transfer, which is also applicable for mild cases of sperm quality and some female issues such as blocked fallopian tubes. In ICSI, the sperm cell is directly injected into the oocyte in vitro, which is mostly used for immotile sperm or immature sperm. It is clearly that these traditional technologies are simple and efficient, without bringing exogenous materials. By contrast, the fabricating of spermbots may be more time-consuming and more expensive. The major disadvantage of spermbots is that it brings the nano components, which may be an unknown risk to the health of both the female recipient and the embryo. Thus, it will be a long-term journey to translate spermbots into in vivo applications for assisted fertilization. Nevertheless, there are also many advantages for spermbots. First, it can be remotely controlled and tracked via magnetic force and ultrasound. Second, the currently available techniques offer multiple options to design various types of spermbots with singular or multiple functionalities, providing comprehensive solutions to solve sperm associated male infertility. Finally, spermbots can also be used to treat gynecologic diseases and tumors in the female reproductive tract.
Correspondingly, we propose a three-step roadmap to translate spermbots into clinical practice (Figure 4C). First, it is foreseeable that in the near future, spermbots could be validated and approved for in vitro clinical usage. Specifically, spermbots can serve as auxiliary or enhanced tools for the current in vitro technologies for assisted fertilization (including IVF and ICSI). For example, the process of sperm cryopreservation will cause a significant decrease of the sperm motility.75 The removable nano motor can be equipped to help the movement and guidance of sperm cells for IVF. However, the technologies of IVF and ICSI may be associated with an increased risk of adverse outcomes such as abortion and preterm birth.76 Thus, we emphasize the first law again: not all sperms are worthy to be rescued by spermbots. Besides genetic diagnosis, the key solutions are to develop advanced and strict sperm selection strategies considering the structural and physicochemical features.77,78 Moreover, the recently emerging artificial intelligence (AI) based tools can also improve the outcomes of assisted fertilization.79 Hence, the combination of in vitro technologies, spermbots, and AI tools will further open a new era for automated, intelligent and precise ART. The second step is to develop drug-loaded spermbots for in vivo treating gynecologic diseases or tumors that occurred in the female reproductive tract. For the preparing of these spermbots, the sperm parts should be edited to lose the ability of fertilizing, or can be fabricated by sperm-like microbots. These spermbots should also be programmed to be site-specific for drug delivery.80 During this stage, the safety and removal of nano components should be carefully evaluated and validated. The final step is to achieve the goal of translating spermbots into in vivo assisted fertilization. Considering the currently available designs of spermbots, the most promising strategy is the recently developed multifunctional spermbots based on 4D-printed microcarriers, which is well designed to be stimuli-responsive to the environment of female reproductive tract.33 However, the safety of the microcarriers and the corresponding controlling and imaging systems should also be validated in vivo in this stage.
Conclusions
The hybrid spermbot is a specialized type of nanobots, which is fabricated by the combination of biological sperm cell and artificial nano component. Previous studies have briefly discussed the possible applications of spermbot in assisted reproduction, which are mostly limited to actuating immotile sperms and targeted drug delivery. Due to the emerging and developing of nanoscale 3D printing technologies, the design and fabrication of spermbots are rapidly evolving in recent years. However, the current development of spermbots is mainly directed by the researchers in the field of nanomaterials. Hence, it is in urgent need to revisit the desired applications in assisted reproduction, from the viewpoints of the researchers and clinicians in the field of reproductive medicine. In the present review, we first summarized and updated the current classification, design, control and application of various spermbots or sperm-like nanobots. Then, we comprehensively summarized the functional features of sperm cells, the journey of sperms to the oocyte, and sperm-related dysfunctions, providing a systematic guidance to further improve the design of spermbots. Although spermbot shows promising applications, it is still staying at the stage of in vitro experiments. We thus pointed out the main challenges of bringing spermbots into clinical practice: biocompatibility, effectiveness of controlling system, and the ethical issues. Considering the special requirements of assisted reproduction, we also proposed the three laws for the clinical applications of spermbots: good genetics, gentle operation and no contamination. Finally, a three-step roadmap was proposed to set up goal-orientated plans for translating spermbots into clinical practice. Although the currently available assisted reproductive technologies, such as IUI, IVF and ICSI, are already simple and efficient, spermbots can further open a new era of automation, intelligence, and precision. Thus, we believe that spermbot-based treatments can be firstly validated and approved for in vitro clinical usage in the near future. However, multi-center and multi-disciplinary cooperation is required to further promote the translation of spermbots into in vivo clinical applications.
Abbreviations
ART, assisted reproductive technologies; IUI, intrauterine insemination; IVF, in vitro fertilization; ICSI, intracytoplasmic sperm injection; WHO, World Health Organization; 3D, three-dimensional; CRISPR, clustered regularly interspaced short palindromic repeats; AI, artificial intelligence; 4D, four-dimensional; DNA, deoxyribonucleic acid; ESHRE, European Society of Human Reproduction and Embryology.
Acknowledgments
This work was supported by the scientific research project of Wuxi Municipal Health Commission (No. M202334) and the top talent support program for young and middle-aged people of Wuxi Health Committee.
Disclosure
The authors report no conflicts of interest in this work.
References
1. Cox CM, Thoma ME, Tchangalova N, et al. Infertility prevalence and the methods of estimation from 1990 to 2021: a systematic review and meta-analysis. Human Reproduct Open. 2022;2022(4):hoac051. doi:10.1093/hropen/hoac051
2. Eisenberg ML, Esteves SC, Lamb DJ, et al. Male infertility. Nature Reviews Disease Primers. 2023;9(1):49. doi:10.1038/s41572-023-00459-w
3. Bhattacharya I, Sharma SS, Majumdar SS. Etiology of Male Infertility: an Update. Reprod Sci. 2023;31:942–965. doi:10.1007/s43032-023-01401-x
4. Boitrelle F, Shah R, Saleh R, et al. The sixth edition of the WHO manual for human semen analysis: a critical review and SWOT analysis. Life. 2021;11(12). doi:10.3390/life11121368
5. Garcia-Grau E, Lleberia J, Costa L, et al. Decline of sperm quality over the last two decades in the South of Europe: a retrospective study in infertile patients. Biology. 2022;12(1):70. doi:10.3390/biology12010070
6. Levine H, Jorgensen N, Martino-Andrade A, et al. Temporal trends in sperm count: a systematic review and meta-regression analysis of samples collected globally in the 20th and 21st centuries. Human Reproduct Update. 2023;29(2):157–176. doi:10.1093/humupd/dmac035
7. Assidi M. Infertility in men: advances towards a comprehensive and integrative strategy for precision theranostics. Cells. 2022;11(10):1711. doi:10.3390/cells11101711
8. Kushnir VA, Barad DH, Albertini DF, Darmon SK, Gleicher N. Systematic review of worldwide trends in assisted reproductive technology 2004–2013. Reprod Biol Endocrinol. 2017;15(1):6. doi:10.1186/s12958-016-0225-2
9. Leusder M, van Elten HJ, Ahaus K, Hilders C, van Santbrink EJP. Protocol for improving the costs and outcomes of assistive reproductive technology fertility care pathways: a study using cost measurement and process mining. BMJ open. 2023;13(6):e067792. doi:10.1136/bmjopen-2022-067792
10. Magdanz V, Sanchez S, Schmidt OG. Development of a sperm-flagella driven micro-bio-robot. Advan Mat. 2013;25(45):6581–6588. doi:10.1002/adma.201302544
11. Lebre F, Chatterjee N, Costa S, et al. Nanosafety: an evolving concept to bring the safest possible nanomaterials to society and environment. Nanomaterials. 2022;12(11). doi:10.3390/nano12111810
12. Singh AV, Ansari MHD, Mahajan M, et al. Sperm cell driven microrobots-emerging opportunities and challenges for biologically inspired robotic design. Micromachines. 2020;11(4):448. doi:10.3390/mi11040448
13. Li J, Dekanovsky L, Khezri B, Wu B, Zhou H, Sofer Z. Biohybrid micro- and nanorobots for intelligent drug delivery. Cyborg Bionic Syst. 2022;2022:9824057. doi:10.34133/2022/9824057
14. Magdanz V, Schmidt OG. Spermbots: potential impact for drug delivery and assisted reproductive technologies. Expert Opin Drug Delivery. 2014;11(8):1125–1129. doi:10.1517/17425247.2014.924502
15. du Plessis SS, Kashou AH, Benjamin DJ, Yadav SP, Agarwal A. Proteomics: a subcellular look at spermatozoa. Reprod Biol Endocrinol. 2011;9:36. doi:10.1186/1477-7827-9-36
16. Medina-Sánchez M, Schwarz L, Meyer AK, Hebenstreit F, Schmidt OG. Cellular cargo delivery: toward assisted fertilization by sperm-carrying micromotors. Nano Lett. 2016;16(1):555–561. doi:10.1021/acs.nanolett.5b04221
17. Xu H, Medina-Sánchez M, Magdanz V, Schwarz L, Hebenstreit F, Schmidt OG. Sperm-hybrid micromotor for targeted drug delivery. ACS nano. 2018;12(1):327–337. doi:10.1021/acsnano.7b06398
18. Magdanz V, Khalil ISM, Simmchen J, et al. IRONSperm: sperm-templated soft magnetic microrobots. Sci Adv. 2020;6(28):eaba5855. doi:10.1126/sciadv.aba5855
19. Middelhoek K, Magdanz V, Abelmann L, Khalil ISM. Drug-Loaded IRONSperm clusters: modeling, wireless actuation, and ultrasound imaging. Biomedical Mat. 2022;17(6). doi:10.1088/1748-605X/ac8b4b
20. Xu H, Medina-Sánchez M, Zhang W, et al. Human spermbots for patient-representative 3D ovarian cancer cell treatment. Nanoscale. 2020;12(39):20467–20481. doi:10.1039/d0nr04488a
21. Zhang Z, Li Z, Yu W, Li K, Xie Z. Development of a biomedical micro/nano robot for drug delivery. J Nanoscie Nanotechnol. 2015;15(4):3126–3129. doi:10.1166/jnn.2015.9643
22. Park D, Park SJ, Cho S, et al. Motility analysis of bacteria-based microrobot (bacteriobot) using chemical gradient microchamber. Biotechnol Bioeng. 2014;111(1):134–143. doi:10.1002/bit.25007
23. Li D, Choi H, Cho S, et al. A hybrid actuated microrobot using an electromagnetic field and flagellated bacteria for tumor-targeting therapy. Biotechnol Bioeng. 2015;112(8):1623–1631. doi:10.1002/bit.25555
24. Ruan J, Kato T, Santini CL, et al. Architecture of a flagellar apparatus in the fast-swimming magnetotactic bacterium MO-1. Proc Natl Acad Sci USA. 2012;109(50):20643–20648. doi:10.1073/pnas.1215274109
25. Ma Q, Chen C, Wei S, Wu LF, Song T, Song T. Construction and operation of a microrobot based on magnetotactic bacteria in a microfluidic chip. Biomicrofluidics. 2012;6(2):24107–2410712. doi:10.1063/1.3702444
26. Chen Y, Kosmas P, Martel S. Microwave breast tumor detection and size estimation using contrast-agent-loaded magnetotactic bacteria. Annu Internat Conf. 2013;2013:5481–5484. doi:10.1109/embc.2013.6610790
27. Khalil ISM, Klingner A, Hamed Y, Magdanz V, Toubar M, Misra S. Characterization of flagellar propulsion of soft microrobotic sperm in a viscous heterogeneous medium. Front Rob AI. 2019;6:65. doi:10.3389/frobt.2019.00065
28. Celi N, Gong D, Cai J. Artificial flexible sperm-like nanorobot based on self-assembly and its bidirectional propulsion in precessing magnetic fields. Sci Rep. 2021;11(1):21728. doi:10.1038/s41598-021-00902-6
29. Mossman JA, Pearson JT, Moore HD, Pacey AA. Variation in mean human sperm length is linked with semen characteristics. Human Reprod. 2013;28(1):22–32. doi:10.1093/humrep/des382
30. Hirano Y, Shibahara H, Shimada K, et al. Accuracy of sperm velocity assessment using the sperm quality analyzer V. Reproduct Med Biol. 2003;2(4):151–157. doi:10.1111/j.1447-0578.2003.00039.x
31. Naser FA, Jaber HA, Rashid MT, Jasim BH. A sperm-based autonomous micro-robot: first step. IFAC-PapersOnLine. 2021;54(17):117–122. doi:10.1016/j.ifacol.2021.11.035
32. Mushtaq F, Torlakcik H, Hoop M, et al. Motile piezoelectric nanoeels for targeted drug delivery. Adv Funct Mater. 2019;29(12):1808135. doi:10.1002/adfm.201808135
33. Rajabasadi F, Moreno S, Fichna K, et al. Multifunctional 4D-printed sperm-hybrid microcarriers for assisted reproduction. Advan Mat. 2022;34(50):e2204257. doi:10.1002/adma.202204257
34. Liu D, Guo R, Wang B, Hu J, Lu Y. Magnetic micro/nanorobots: a new age in biomedicines. Adv Intell Sys. 2022;4(12):2200208. doi:10.1002/aisy.202200208
35. Wang Q, Zhang L. Ultrasound imaging and tracking of micro/nanorobots: from individual to collectives. IEEE Open J Nanotechnol. 2020;1:6–17. doi:10.1109/ojnano.2020.2981824
36. Deng Y, Paskert A, Zhang Z, Wittkowski R, Ahmed D. An acoustically controlled helical microrobot. Sci Adv. 2023;9(38):eadh5260. doi:10.1126/sciadv.adh5260
37. Magdanz V, Guix M, Hebenstreit F, Schmidt OG. Dynamic polymeric microtubes for the remote-controlled capture, guidance, and release of sperm cells. Advan Mat. 2016;28(21):4084–4089. doi:10.1002/adma.201505487
38. Paxton WF, Kistler KC, Olmeda CC, et al. Catalytic nanomotors: autonomous movement of striped nanorods. J Am Chem Soc. 2004;126(41):13424–13431. doi:10.1021/ja047697z
39. Calvo-Marzal P, Sattayasamitsathit S, Balasubramanian S, Windmiller JR, Dao C, Wang J. Propulsion of nanowire diodes. Chem Communicat. 2010;46(10):1623–1624. doi:10.1039/b925568k
40. Palagi S, Mark AG, Reigh SY, et al. Structured light enables biomimetic swimming and versatile locomotion of photoresponsive soft microrobots. Nature Mater. 2016;15(6):647–653. doi:10.1038/nmat4569
41. Dcunha R, Hussein RS, Ananda H, et al. Current insights and latest updates in sperm motility and associated applications in assisted reproduction. Reprod Sci. 2022;29(1):7–25. doi:10.1007/s43032-020-00408-y
42. Oren-Benaroya R, Orvieto R, Gakamsky A, Pinchasov M, Eisenbach M. The sperm chemoattractant secreted from human cumulus cells is progesterone. Human Reprod. 2008;23(10):2339–2345. doi:10.1093/humrep/den265
43. Boryshpolets S, Pérez-Cerezales S, Eisenbach M. Behavioral mechanism of human sperm in thermotaxis: a role for hyperactivation. Human Reprod. 2015;30(4):884–892. doi:10.1093/humrep/dev002
44. Fitzpatrick JL, Willis C, Devigili A, et al. Chemical signals from eggs facilitate cryptic female choice in humans. Proc Biol Sci. 2020;287(1928):20200805. doi:10.1098/rspb.2020.0805
45. Zhang Z, Liu J, Meriano J, et al. Human sperm rheotaxis: a passive physical process. Sci Rep. 2016;6:23553. doi:10.1038/srep23553
46. Ahmadkhani N, Saadatmand M, Kazemnejad S, Abdekhodaie M. Qualified sperm selection based on the rheotaxis and thigmotaxis in a microfluidic system. Biomed Eng Lett. 2023;13(4):671–680. doi:10.1007/s13534-023-00294-8
47. Parker GA. Conceptual developments in sperm competition: a very brief synopsis. Philos Trans R Soc London, Ser B. 2020;375(1813):20200061. doi:10.1098/rstb.2020.0061
48. Amaral A, Herrmann BG. RAC1 controls progressive movement and competitiveness of mammalian spermatozoa. PLoS genetics. 2021;17(2):e1009308. doi:10.1371/journal.pgen.1009308
49. Fisher HS, Hoekstra HE. Competition drives cooperation among closely related sperm of deer mice. Nature. 2010;463(7282):801–803. doi:10.1038/nature08736
50. Ishimoto K, Gaffney EA. Hydrodynamic Clustering of Human Sperm in Viscoelastic Fluids. Sci Rep. 2018;8(1):15600. doi:10.1038/s41598-018-33584-8
51. Primakoff P, Myles DG. Penetration, adhesion, and fusion in mammalian sperm-egg interaction. Science. 2002;296(5576):2183–2185. doi:10.1126/science.1072029
52. Shivalkar S, Roy A, Chaudhary S, Samanta SK, Chowdhary P, Sahoo AK. Strategies in design of self-propelling hybrid micro/nanobots for bioengineering applications. Biomedical Mat. 2023;18(6). doi:10.1088/1748-605X/acf975
53. Gupta A, Soni S, Chauhan N, Khanuja M, Jain U. Nanobots-based advancement in targeted drug delivery and imaging: an update. J Controll Rel. 2022;349:97–108. doi:10.1016/j.jconrel.2022.06.020
54. Smith LB, Walker WH. The regulation of spermatogenesis by androgens. Semin Cell Dev Biol. 2014;30:2–13. doi:10.1016/j.semcdb.2014.02.012
55. James ER, Carrell DT, Aston KI, Jenkins TG, Yeste M, Salas-Huetos A. The role of the epididymis and the contribution of epididymosomes to mammalian reproduction. Int J Mol Sci. 2020;21(15):5377. doi:10.3390/ijms21155377
56. Suarez SS, Pacey AA. Sperm transport in the female reproductive tract. Human Reproduct Update. 2006;12(1):23–37. doi:10.1093/humupd/dmi047
57. Sharma A, Minhas S, Dhillo WS, Jayasena CN. Male infertility due to testicular disorders. J Clin Endocrinol Metab. 2021;106(2):e442–e459. doi:10.1210/clinem/dgaa781
58. Parekh NV, Lundy SD, Vij SC. Fertility considerations in men with testicular cancer. Transl Androlo Urol. 2020;9(Suppl 1):S14–S23. doi:10.21037/tau.2019.08.08
59. Ibtisham F, Honaramooz A. Spermatogonial stem cells for in vitro spermatogenesis and in vivo restoration of fertility. Cells. 2020;9(3):745. doi:10.3390/cells9030745
60. Robinson M, Sparanese S, Witherspoon L, Flannigan R. Human in vitro spermatogenesis as a regenerative therapy – where do we stand? Nat Rev Urol. 2023;20(8):461–479. doi:10.1038/s41585-023-00723-4
61. Lee RG, Mazzola AM, Braun MC, et al. Efficacy and safety of an investigational single-course CRISPR base-editing therapy targeting PCSK9 in nonhuman primate and mouse models. Circulation. 2023;147(3):242–253. doi:10.1161/circulationaha.122.062132
62. Goldsmith T, Bondareva A, Webster D, et al. Targeted gene editing in porcine germ cells. Meth Molecul Biol. 2022;2495:245–258. doi:10.1007/978-1-0716-2301-5_13
63. Colpi GM, Francavilla S, Haidl G, et al. European Academy of Andrology guideline Management of oligo-astheno-teratozoospermia. Andrology. 2018;6(4):513–524. doi:10.1111/andr.12502
64. Shibahara H, Wakimoto Y, Fukui A, Hasegawa A. Anti-sperm antibodies and reproductive failures. Am J Reproduct Immunol. 2021;85(4):e13337. doi:10.1111/aji.13337
65. Agarwal A, Aponte-Mellado A, Premkumar BJ, Shaman A, Gupta S. The effects of oxidative stress on female reproduction: a review. Reprod Biol Endocrinol. 2012;10:49. doi:10.1186/1477-7827-10-49
66. Gholiof M, Adamson-De Luca E, Wessels JM. The female reproductive tract microbiotas, inflammation, and gynecological conditions. Front Reprod Health. 2022;4:963752. doi:10.3389/frph.2022.963752
67. Jain K, Shukla R, Yadav A, Ujjwal RR, Flora SJS. 3D Printing in Development of Nanomedicines. Nanomaterials. 2021;11(2). doi:10.3390/nano11020420
68. Jans V, Dondorp W, Goossens E, Mertes H, Pennings G, de Wert G. Balancing animal welfare and assisted reproduction: ethics of preclinical animal research for testing new reproductive technologies. Med Health Care Philos. 2018;21(4):537–545. doi:10.1007/s11019-018-9827-0
69. Li J, Yu J. Biodegradable microrobots and their biomedical applications: a Review. Nanomaterials. 2023;13(10). doi:10.3390/nano13101590
70. Borges ED, Berteli TS, Reis TF, Silva AS, Vireque AA. Microbial contamination in assisted reproductive technology: source, prevalence, and cost. J Ass Reprod Genet. 2020;37(1):53–61. doi:10.1007/s10815-019-01640-5
71. AS V, Dhama K, Chakraborty S, et al. Role of antisperm antibodies in infertility, pregnancy, and potential for contraceptive and antifertility vaccine designs: research progress and pioneering vision. Vaccines. 2019;7(3). doi:10.3390/vaccines7030116
72. Londra L, Wallach E, Zhao Y. Assisted reproduction: ethical and legal issues. Semin Fetal Neonatal Med. 2014;19(5):264–271. doi:10.1016/j.siny.2014.07.003
73. Almeida M, Ranisch R. Beyond safety: mapping the ethical debate on heritable genome editing interventions. Humanit Soc Sci Commun. 2022;9(1):139. doi:10.1057/s41599-022-01147-y
74. Cissen M, Bensdorp A, Cohlen BJ, Repping S, de Bruin JP, van Wely M. Assisted reproductive technologies for male subfertility. Cochrane Database Syst Rev. 2016;2(2):CD000360. doi:10.1002/14651858.CD000360.pub5
75. Wang J, Wang M, Hong R, et al. Quantitative phosphoproteomics reveals GSK3A substrate network is involved in the cryodamage of sperm motility. Biosci Rep. 2021;41(10). doi:10.1042/bsr20211326
76. Li J, Shen J, Zhang X, et al. Risk factors associated with preterm birth after IVF/ICSI. Sci Rep. 2022;12(1):7944. doi:10.1038/s41598-022-12149-w
77. Ribas-Maynou J, Barranco I, Sorolla-Segura M, Llavanera M, Delgado-Bermúdez A, Yeste M. Advanced sperm selection strategies as a treatment for infertile couples: a systematic review. Int J Mol Sci. 2022;23(22):13859. doi:10.3390/ijms232213859
78. Zhu Y, Zhang F, Cheng H, Sun XX, Jiang F. Modified strict sperm morphology threshold aids in the clinical selection of conventional in vitro fertilization (IVF) or intracytoplasmic sperm injection (ICSI). As J Androl. 2022;24(1):62–66. doi:10.4103/aja.aja_45_21
79. Lustgarten Guahmich N, Borini E, Zaninovic N. Improving outcomes of assisted reproductive technologies using artificial intelligence for sperm selection. Fertil Sterility. 2023;120(4):729–734. doi:10.1016/j.fertnstert.2023.06.009
80. Cheng R, Meng F, Deng C, Klok HA, Zhong Z. Dual and multi-stimuli responsive polymeric nanoparticles for programmed site-specific drug delivery. Biomaterials. 2013;34(14):3647–3657. doi:10.1016/j.biomaterials.2013.01.084