Tech
Antimicrobial peptides effects on klebsiella pneumoniae | IDR
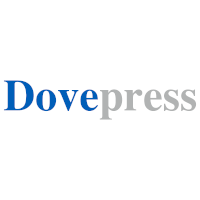
Xiaochun Chen,1,* Benhong Zhang,2,* Jin He,3,* Xiaohong Rui,4 Tian He,4 Lizhu Zhang,5 Junfeng Bao,6 Yanfei Jing,7 Futao Cao8
1Department of Laboratory Medicine, Taizhou Second People’s Hospital, Taizhou, People’s Republic of China; 2Department of Laboratory Medicine, Hangzhou Gongshu District Integrated Traditional Chinese and Western Medicine Hospital, Hangzhou, People’s Republic of China; 3Department of Laboratory Medicine, Hangzhou Yuhang Jiamu Nursing Home, Hangzhou, People’s Republic of China; 4Department of Laboratory Medicine, Affiliated Wuxi Fifth Hospital of Jiangnan University, Wuxi, People’s Republic of China; 5Department of Research, Nanxin Pharm, Nanjing, People’s Republic of China; 6Department of Laboratory Medicine, Wuxi Maternal and Child Health Care Hospital, Women’s Hospital of Jiangnan University, Wuxi, People’s Republic of China; 7Department of Function, Affiliated Wuxi Fifth Hospital of Jiangnan University, Wuxi, People’s Republic of China; 8Department of Emergency, Jiangnan University Medical Center, Wuxi, People’s Republic of China
Correspondence: Yanfei Jing, Department of Function, Affiliated Wuxi Fifth Hospital of Jiangnan University, Wuxi, Jiangsu, 214005, People’s Republic of China, Email [email protected] Futao Cao, Department of Emergency, Jiangnan University Medical Center, Wuxi, Jiangsu, 214000, People’s Republic of China, Email [email protected]
Introduction: The emergence of multidrug-resistant Klebsiella pneumoniae (K. pneumoniae) and the decline of effective antibiotics lead to the urgent need for new antibacterial agents. The aim of this study is to investigate the therapeutic effect of antimicrobial peptides against gentamicin-resistant (RT) K. pneumoniae and to screen effective antimicrobial peptides.
Methods: In this study, the RT strains were induced by gradient gentamicin, and the RT strains were selected by detecting the expression levels of efflux pump genes, porin genes, and biofilm formation genes of the strains combined with their effects on the cells. Then the effects of four antimicrobial peptides on the efflux pump activity, biofilm formation level and cell condition after infection were detected to explore the effects of antimicrobial peptides on RT strains. Finally, the RT strain was used to induce a mouse model of pneumonia, and the four antimicrobial peptides were used to treat pneumonia mice for in vivo experiments. The pathological changes in lung tissues in each group were detected to explore the antimicrobial peptide with the most significant effect on the RT strain in vivo.
Results: The results showed that the minimal inhibitory concentrations of the RT strains (strain C and strain I) were significantly higher than those of the wild-type strain, and the expression of efflux pump, porin and biofilm formation genes was significantly increased. The antimicrobial peptides could effectively inhibit the biofilm formation and efflux pump protein function of the RT strains. In addition, the antimicrobial peptides showed promising antibacterial effects both in vitro and in vivo.
Discussion: Our study provided a theoretical basis for the treatment of gentamicin resistant K. pneumoniae infection with antimicrobial peptides, and found that KLA was significantly superior to LL37, Magainin I, KLA and Dermaseptin (10 μg/mL in cells, 50 μg in mice).
Keywords: Klebsiella pneumoniae, K. pneumoniae, antibiotics, antimicrobial peptide, gentamicin-resistant, RT, microenvironment
Introduction
As a gram-negative bacterium with opportunistic pathogenicity, Klebsiella pneumoniae (K. pneumoniae) is the leading cause of hospital-acquired infections (HAIS),1,2 such as urinary tract infection, pneumonia and bloodstream infection in hospitalized or other immunocompromised patients.3–6 In 2019, the global death toll caused by and related to antimicrobial drug resistance of K. pneumoniae was approximately 200,000 to 650,000 cases, ranking third among the death tolls caused by drug resistance of various antibacterial drugs.7 The mortality rate of hypervirulent K. pneumoniae in endemic areas is 12% to 45%.8 Moreover, the isolation rate of K. pneumoniae in China is also increasing year by year.9 At present, carbapenems, β-lactams, quinolones, aminoglycosides and other drugs are commonly used for the treatment of K. pneumoniae infection.10 However, due to the abuse of antimicrobial drugs, multidrug-resistant strains have appeared in K. pneumoniae, which has aroused great clinical and social attention.1,11 The resistance mechanism of K. pneumoniae mainly includes (1) producing enzymes to inactivate antibiotics;12 (2) change in target sites of antimicrobial agents;13 (3) decrease in drug penetration;14 (4) overexpression of efflux pump gene.15 Among them, increasing efflux by efflux pumps (EPs) plays an important role in the multidrug resistance of bacteria.16,17
Gentamicin, one of the highly effective broad-spectrum aminoglycosides antibiotics active against various Bacilli, Pseudomonas aeruginosa and Gram-positive cocci, has been used in the clinical treatment of bacterial infections.18,19 Like other aminoglycosides, gentamicin exerts antimicrobial effects by interfering with bacterial protein synthesis. Gentamicin can bind 16S rRNA on the 30S subunit of bacterial ribosomes, interfering with the attachment of formyl-methionyl-tRNA to 30S rRNA and blocking bacterial protein synthesis.20 Due to its wide application, the increasing resistance of bacteria to gentamicin poses a challenge to human health.3 Moreover, the clinical application of gentamicin is limited by its significant nephrotoxic and neurotoxic effects.21
Antimicrobial peptides (AMP), a type of small-molecule weight short peptides in organisms, are generated by specific gene transcription.22 They are active molecules generated and retained during biological evolution. With broad antibacterial and antiviral activities, antimicrobial peptides regulate innate immunity, clear mutant cells, and are essential to the biological innate immune system.23–26 Increasing attention has been attracted to the research of antimicrobial peptides for clinical application due to their diverse bactericidal mechanisms, biological activity and excellent properties, and inhibition of bacteria resistance to various antibiotics.27
LL37, the only known human antimicrobial peptide (CAMP), as an endogenous host defense peptide, has attracted a lot of research attention due to its broad-spectrum antibacterial activity and immunomodulatory properties,28 and is considered as a promising choice for the treatment of biomembrane-associated infectious diseases, which can prevent K. pneumoniae from forming biofilms by down-regulating biofilm-related genes and degrading biofilm matrix29 and has great potential as an antibiotic against carbapenem-resistant K. pneumoniae.30
Magainin I is an antimicrobial peptide that specifically interacts with bacteria.31 The results of Mayram Hacioglu et al showed that magainin (100µg/mL) was ineffective against K. pneumonia,32 while Laura Bedin Denardi et al showed that magainin-2 (80–160 mg/L) had strong bactericidal activity against carbapenem-resistant K. pneumoniae.30
KLA is an antimicrobial peptide that is modified to form a cyclic structure to limit helicity and thus reduce cytotoxicity,33 and KLA mediated by neutrophil membrane-coated nanoparticles (KLA-Nnps) was found to be effective in inhibiting the development of pneumonia caused by drug-resistant K. pneumonia.34
Dermaseptins, a type of α-helical polycationic peptides isolated from Hylid frogs, have antibacterial effects against bacteria, parasites, protozoa and viruses in vitro. In addition, a 10-peptide derivative of the N-terminal domain of Dermaseptin-PC showed significant antimicrobial activity against K. pneumonia and showed synergistic antimicrobial activity with gentamicin against carbapenem-resistant K. pneumonia.35
All the above four antimicrobial peptides have a certain effect on K. pneumonia resistance, but the effect on gentamicin-resistant K. pneumonia has not been studied. In this study, ten K. pneumoniae strains isolated from patients were screened with gentamicin at gradient concentrations to obtain drug-resistant strains. Therefore, in this study, 10 strains of K. pneumoniae isolated from patients were screened by gradient concentration of gentamicin to obtain drug-resistant strains, and their efflux pump activity and biofilm formation ability were detected to analyze the reasons for drug resistance of K. pneumonia, and the effects and differences of antimicrobial peptides LL37, Magainin I, KLA and Dermaseptin against K. pneumoniae infection in vitro and in vivo were studied.
Materials and Methods
Bacterial Strains and Reagents
A total of ten K. pneumoniae strains (A-J) were obtained from the biological samples (including blood, throat, rectum, and catheter) of the patients in Wuxi Fifth People’s Hospital, from January 2014 to June 2020. This study was approved by the Ethics Committee of our hospital (No. 2021–021-1). All strains were identified using the VITEK 2 system (bioMérieux, France). The resistance profile of the strains used in this study is presented in Table 1. The standard strain K. pneumoniae ATCC13883 was purchased from the Clinical Laboratory Center of the Ministry of Health (Beijing, China). Mueller–Hinton broth, MH agar, Luria-Bertani (LB) broth, carbonyl cyanide m-chlorophenylhydrazone (CCCP), ethidium bromide (EtBr), sodium acetate, crystal violet and phosphate buffered saline (PBS) were purchased from Sigma-Aldrich (Darmstadt, Germany). Antibacterial AgNPs were obtained from Yamei nanotech (Zhejiang, China). Antimicrobial peptides (LL37, Dermaseptin, Magainin 1 and KLA) were designed and synthesized by China Peptides Co., Ltd (Shanghai, China). Mouse TNF-α, IL-1β and IL-6 ELISA kit, Hematoxylin and Eosin (HE) Staining Kit and DAB (Diaminobenzidine) Horseradish Peroxidase Color Development Kit were purchased from Beyotime (Shanghai, China). Anti-Ly6G antibody (ab261916) and Goat Anti-Rabbit IgG H&L (HRP) (ab6721) were purchased from Abcam (Cambridge, England).
Table 1 The Antibiotic Resistance Profile of Klebsiella pneumonia |
Cell Culture
Human lung cell line (HLF-a) was purchased from Procell Life Science & Technology Co., Ltd (Wuhan, China). Cells were cultured in Dulbecco’s modified Eagle’s medium (DMEM) (Yeasen, Shanghai, China) containing 10% fetal bovine serum (FBS, Sigma-Aldrich, Darmstadt, Germany) with 1% Penicillin/Streptomycin in a humidified incubator containing 5% CO2 at 37°C.36
Drug-Resistant Strain Induction
The K. pneumoniae strains (A-J) were inoculated on fresh agar plates for 18~24 h at 37°C. A single colony was selected and inoculated in 10 mL LB liquid medium for oscillating culture for 18 h. The supernatant was removed by centrifugation. The bacteria solution was resuspended with sterile normal saline and adjusted to 1.5 × 108 CFU/mL. Then, 150 μL of bacteria solution was evenly spread on the surface of the Mueller–Hinton (MH) agar plate containing gentamicin (concentration of 0.5~256 mg/L). After being incubated in an incubator at 37°C for 3 days, the colonies on the plate were picked up and adjusted to 1.5 × 108 CFU/mL.
Similarly, 150 μL of bacteria solution was evenly spread on the next gradient concentration plate for further induction, gradient by the gradient. After incubation for four weeks, the colony number in each gradient was calculated, and the monoclonal was screened out for further incubation in LB medium with corresponding concentrations of gentamicin. The drug-resistant strains were obtained in the 10th generation of induction, respectively. Drug susceptibility test and PCR analysis were performed for the expression of efflux pump genes, porin genes and biofilm formation genes to verify the resistant strains.37
Cytotoxicity Assay
The toxicity of the antimicrobial peptides to HLF-a cells was detected by the release of lactate dehydrogenase (LDH).38 HLF-a cells were grown in 96-well plates and cultured overnight. Subsequently, 100 μL of the peptides (LL37, Magainin I, KLA and Dermaseptin; concentration range: 1000–0.98 μg/mL) diluted in DMEM was added into the plates and incubated at 37°C for 24 h.39,40 The control group was cultured in DMEM without peptides or in medium with 0.5% Triton X-100 (Thermo Scientific, Shanghai, China). The plates were added with 50 μL of LDH reaction mixture (Pierce, Rockford, IL, USA) and cultured for 30 min at room temperature. Next, each well was added with 50 μL stop solution. Finally, the OD values at 490 nm were measured for LDH activity using a microplate reader (BioTek, Biotek Winooski, VT, USA).
Detection of the Drug Resistance and Biofilm Forming Genes
The mRNA levels of gentamicin-resistance related genes41 (acrA, acrB, tolC, acrR, ompK35 and ompK36) and biofilm forming genes42 (mrkA, luxS, pga, wbbM, and wzm) of K. pneumoniae were detected by real-time PCR (RT-PCR). In brief, the genomic DNA of K. pneumoniae, as the template for PCR reaction, was extracted according to the instructions of the Bacterial Genomic DNA Extraction Kit (Solarbio, Beijing, China). The concentration and quality of RNA were measured using a spectrophotometer. Then 2 μg of total RNA was reverse transcribed into cDNA using a PrimeScript RT reagent kit (TaKaRa, Dalian, China). RT-qPCR was performed using a SYBR Green PCR Kit (Vazyme, Nanjing, China) on ABI 7500 Rapid real-time PCR system (Applied Biosystems, Foster City, CA, USA). The thermal cycle consisted of reverse transcription at 42°C for 5 min, initial denaturation at 95°C for 10s, 45 cycles of denaturation at 95°C for 5 s, and annealing/extension at 55°C for 10s. The mRNA expression of acrA, acrB, tolC, acrR, ompK35 and ompK36 gene was normalized by the expression of 16S rRNA. The quantitative analysis was obtained using the 2−ΔΔCT method.43 The primers used in the study are listed in Table 2.
![]() |
Table 2 Primer Sequences Used for RT-PCR |
Minimal Inhibitory Concentration (MIC) Assay
The minimal inhibitory concentration (MIC) of the 10 strains for gentamicin was detected by the two-fold broth microdilution method in 96-well microtiter plates according to the method recommended by the Clinical and Laboratory Standards Institute (CLSI, 2021). Briefly, the bacterial colonies were suspended and grown in Muller Hinton broth. The suspension was adjusted to McFarland standard 0.5 to obtain a concentration of 105 CFU/mL. An equal amount of 0.05 mL was transferred to each well of a 96-well plate containing 0.05 mL gentamicin at a concentration twice as diluted as that continuously prepared in a nutrient solution medium. After the incubation at 37°C for 16–18 h, MIC results were recorded. The experiments were performed three times.22
Biofilm Assay
K. pneumoniae were cultured in LB broth overnight and diluted to a density of 1 × 107 CFU/mL. 100 μL of the diluted bacteria were added to the 96-well microtiter plate containing gentamicin and 10 μg/mL antimicrobial peptides (LL37, Magainin I, KLA and Dermaseptin) and inoculated for 24 h at 37°C.44,45 The solution was removed, and the wells were washed with PBS thrice to remove the bacteria. The biofilm was fixed with sodium acetate and then stained with crystalline violet (0.1%) for 10 min. The plates were rinsed to remove excess dye and dried. The combined crystalline violet was then dissolved by 200 μL of 95% ethanol. Furthermore, the A570nm was measured on a microplate spectrophotometer (Biotek, Biotek Winooski, VT, USA). Moreover, the %age biofilm inhibition of gentamicin treated experiment was calculated as follows: %age biofilm inhibition = 1- OD570 of cells treated with Gentamicin/OD570 of nontreated cells × 100.2,46
Ethidium Bromide (EtBr) Accumulation Assay
As previously described, ethidium bromide (EtBr) was used to evaluate the efflux capacity of strains.2 Briefly, K. pneumoniae strains were cultured in nutrient broth until the logarithmic growth phase. The bacteria were collected and washed with 1 × PBS 3 times. The bacteria were then resuspended and OD600 was adjusted to 0.3. Next, 200 μL of bacterial solution was added to a 96-well black plate. EtBr (50 μM) and the CCCP (100 μM) or antimicrobial peptides (LL37, Magainin I, KLA and Dermaseptin; 10 μg/mL) were added to each well and incubated at 37°C for 30 min with 5% glucose. Each sample was set to three repeats. The light was avoided during the experiment. After adding EtBr, fluorescence detection was performed immediately using a Multiskan MK3 multifunctional enzyme plate analyzer (Thermo Fisher Scientific, Waltham, MA, USA), measured every 2 min for a total duration of 30 min. The excitation wavelength was set at 530 nm, and the emission spectrum was set at 585 nm.
Fluorescence Detection
Logarithmic phase cells (1 × 105 cells/well) were inoculated in 6-well Chamber Slide (Thermo fisher Scientific, Waltham, MA, USA), incubated with buffer mixed with Klebsiella pneumoniae for 24 h. After washing twice with PBS, lung cells were fixed on ice with 4% paraformaldehyde for 15 min, then infiltrated with 0.5% TritonX-100 at room temperature for 30 min. Gentamicin (32 mg/L) and 10 μg/mL antimicrobial peptides (LL37, Magainin I, KLA and Dermaseptin) were treated in different groups for 1 h. Next, lung cells infected with Klebsiella pneumoniae were incubated with hybridization buffer mixed with fluorescence in situ hybridization (FISH) probe (Ribobio, red, Guangzhou, China). The nuclei were stained (blue) with 6-diamino-2-phenylindole (DAPI). Lung cells infected with Klebsiella pneumoniae were observed under a confocal fluorescence microscope (Thermo Fisher Scientific, Waltham, MA, USA).47,48
Flow Cytometry
The cell apoptosis was detected by flow cytometry analysis. In brief, the K. pneumoniae-infected HLF-a cells at the logarithmic growth stage (4 × 106 /mL) were inoculated into a 6-well plate, and 80 μM of RES containing 10% fetal bovine serum was added and cultured at 37°C and 5% CO2. After 48 h, the cells were collected and then suspended in 195 μL of binding buffer (1 × 106 cells/mL). The cells were then incubated with 5 μL of AnnexinV-Alexa Fluor 647 (Alexa Fluor® 647, 1:200, Abcam, Cambridge, England) for 15 min and then co-incubated with 5 μL of PI at room temperature. FITC+ and PI− cells were defined as apoptotic cells. Finally, the apoptosis rate was measured using a flow cytometer (Beckman Coulter, CA, USA).49
Animals
Specific-pathogen-free (SPF) grade 6–8 weeks old C57BL/6 mice were purchased from Shanghai Laboratory Animal Centre (Shanghai, China). All mice were routinely raised in the Animal Experiment Center of Jiangnan University. The experimental scheme was approved by the Animal Ethics Committee of the Jiangnan University. After one week of adaptive feeding, we anesthetized mice using 1–1.5% isoflurane inhalation method (IsoFlo, Abbott Laboratories) for 2–3 min and intubated them endotracheally using a blunt tip feeding needle. The 50 μL of K. pneumoniae solution (concentration: 1 × 106 CFU/mL) was administered by blunt needle inoculation. After 6 h of infection, the mice were divided into 6 groups randomly (n = 6 for each group). The mice were then injected via the tail vein with gentamicin (1.5 mg/kg), 50 μg of antimicrobial peptides (LL37, Magainin I, KLA and Dermaseptin) or an equivalent volume of saline for infected mice, respectively. The mice were euthanized with 1–1.5% isoflurane at 16 h or 48 h after treatment, and the lung tissues were collected50,51 after confirmation of death. Under aseptic conditions, the lungs of each group of mice were ground into homogenate and diluted 100 times with sterile PBS. Then, 100 μL of the homogenate was spread onto MH agar plates and incubated at 37°C. The colonies were counted after 24h.
Detection of the Cytokines in Lung
The lung tissue homogenate of the mice sacrificed 48h after the induction of pneumonia was repeatedly frozen and thawed twice, and then centrifuged at 5000 g for 5 min at 4°C to collect the supernatant.46 The corresponding ELISA kit (MultiSciences, Beijing, China) was used to detect the cytokines TNF-α, IL-1β and IL-6 in the supernatant. The samples and standards were added to the antibody-coated plates, and the biotinylated antibody was added after incubation. The HRP-labeled streptavidin was added after binding, and the color development was conducted with tetramethylbenzidine (TMB). The A450 was immediately detected after adding the stop solution. The concentration of cytokines in the sample was calculated according to the standard curve.
Pathological Analysis of Lung Tissue
For hematoxylin-eosin (HE) staining, lung tissues collected from the six mouse groups were fixed with 10% formalin and embedded in paraffin. Then, paraffin sections (4 μm) were stained with hematoxylin and eosin to observe lung tissue lesions. Ly-6G is a neutrophil antigen, and the neutrophils are bactericidal. The immunohistochemical staining was performed to observe the expression of Ly-6G. In short, after dewaxing and hydration, the endogenous peroxidase activity was inactivated. The antigen was retrieved, and then the slices were incubated with Ly-6G monoclonal antibody (1/2000, Abcam, Cambridge, England). Next, HRP-labeled secondary antibody (1/2000, Abcam, Cambridge, England), and finally, color development was conducted with DAB-H2O2.
Statistical Analysis
Data are expressed as the means ± standard deviation of the mean (SD). All experiments were carried out three times independently. Comparisons between the untreated control and treated groups were analyzed with the one-way ANOVA followed by the Bonferroni post-hoc test. A P-value of
Results
This study aimed to investigate the effect of the antimicrobial peptide on the resistance of K. pneumoniae to gentamicin. The gentamicin-resistant strains were screened in this study. We hypothesized that the drug resistance of K. pneumoniae was caused by the enhancement of efflux pump activity and biofilm forming ability of K. pneumoniae. We also investigated the effects of antimicrobial peptides on Klebsiella pneumoniae induced infection in vitro and in vivo.
Screening and Identification of the Drug-Resistant K. Pneumoniae Strains
The antimicrobial susceptibility of the K. pneumoniae collection against gentamicin based on MIC values for each strain before and after induction was depicted in Figure 1A and B. Before induction, all wild-type isolates (A-J) were phenotypically susceptible to gentamicin (MICFigure 1A). However, after gentamicin induction, among the ten K. pneumoniae isolates, five induced strains were found to be gentamicin-resistant (MIC>5 mg/L), especially in C and I isolate (MIC>10 mg/L) (Figure 1B). Therefore, gentamicin-resistant strains C and I were chosen for subsequent experiments. To testify the resistance of K. pneumoniae, we detected the mRNA levels of efflux pump genes (acrA, acrB, and tolC), the porin genes (OmpK35 and OmpK36) and pump regulators (acrR) by RT-PCR. Compared with the wild-type isolates (WT), the expression of OmpK35, OmpK36, acrA, acrB, acrR and tolC genes was significantly increased in the gentamicin-resistance (RT) strains C and I (Figure 1C, PK. pneumoniae strains by ethidium bromide (EtBr). In the absence of efflux pump inhibitor CCCP, all strains had roughly the same fluorescence value, which suggested that the efflux capacity of all strains was similar. However, the fluorescence value of strains with CCCP was significantly higher than that of strains without CCCP, which revealed that EtBr accumulation was increased in the presence of CCCP. Moreover, the fluorescence value of RT strains was lower than that of WT strains in the presence of CCCP (Figure 1D).
Evaluation of Biofilm-Forming Ability of Drug-Resistant K. Pneumoniae Strains
In order to explore whether the drug resistance of RT strains C and I was mediated by the formation of biofilms, we used RT-PCR to detect the mRNA levels of biofilm-forming genes (mrkA, luxS, pga, wbbM, and wzm). Compared with the WT isolates, the expressions of mrkA, luxS, pga, wbbM, and wzm genes were all up-regulated in RT strains C and I (Figure 2A, PFigure 2B, P
Antibacterial Effect of Antibacterial Peptides in vitro
The toxicity of antibacterial peptides to lung cells was first investigated. As shown in Figure 3A, the LL37, KLA and Dermaseptin reached 50% release of LDH at nearly 0.5 mg/mL, while the LD50 for Magainin I was above 1 mg/mL. We then investigated the inhibitory effects of the antimicrobial peptides LL37, Magainin I, KLA and Dermaseptin on the biofilm formation of RT strains C and I. Compared with the negative control group, the treatment of antimicrobial peptides had a significant inhibitory effect on the biofilm formation of the RT strains C and I. Among the four antimicrobial peptides, KLA had the strongest inhibition ability for biofilm formation, which was superior to gentamicin (Figure 3B and C, PFigure 3D and E, the fluorescence value was similar in the PBS and gentamicin groups of strains C and I. However, in strain C, LL37, Magainin I, KLA and Dermaseptin groups showed significantly increased fluorescence values, while in strain I, only Magainin I, KLA and Dermaseptin groups showed significantly increased fluorescence value. For strain C and strain I, the fluorescence accumulation in the KLA treatment group was the highest. This result demonstrated that antimicrobial peptides inhibited the efflux activity of the RT strains.
Antimicrobial Peptides Inhibit the Infection of K. pneumoniae on Lung Cells
To further investigate the effects of antimicrobial peptides (LL37, Magainin I, KLA and Dermaseptin) on RT K. pneumoniae, fluorimetry was performed in lung cells infected with RT strain C then treated with antimicrobial peptides including LL37, KLA, Magainin and Dermaseptin. As shown in Figure 4A, no significant difference was observed between PBS and Gentamicin group, which indicated that K. pneumoniae strain C developed resistance to gentamicin. Compared with PBS and Gentamicin, four antimicrobial peptides significantly reduced the red fluorescence in K. pneumoniae-infected lung cells, especially in KLA (P K. pneumoniae. Furthermore, flow cytometry results showed that annexin V positive cell density in LL37, KLA and Dermaseptin groups was lower than that in PBS or Gentamicin groups. At the same time, Magainin I had no significant effect on K. pneumoniae-infected lung cells (Figure 4B, P K. pneumoniae strain C developed resistance to gentamicin, and the antimicrobial peptides including LL37, KLA and Dermaseptin can effectively inhibit the increased annexin V positive cells caused by K. pneumoniae infection and resistance to gentamicin.
Antibacterial Effect of Antibacterial Peptides in vivo
In order to investigate the inhibition of antimicrobial peptides on RT K. pneumoniae in vivo, we used RT strain C to induce pneumonia in mice. The four kinds of antimicrobial peptides were used to treat pneumonia mice, and the therapeutic effect was then evaluated. As shown in Figure 5A, after antimicrobial peptide treatment for 16 h or 48 h, the bacterial burden in the lungs of mice was significantly decreased in the LL37, KLA, Magainin I and gentamicin groups compared with that in the control group (PPFigure 5B, P Figure 5C). Immunohistochemical staining of the neutrophil antigen LY6G in lung tissue indicated that the antimicrobial peptides reduced the accumulation of neutrophils in lung tissue (Figure 5D).
Discussion
In the present study, we aimed to study the effects of multiple antimicrobial peptides on gentamicin-resistant K. pneumoniae and K. pneumoniae-infected lung cells so as to discover the dominant antimicrobial peptides for the treatment of gentamicin-resistant K. pneumoniae. Our results demonstrated that antimicrobial peptides (LL37, Magainin I, KLA and Dermaseptin) potentially exerted antimicrobial effects by inhibiting efflux activity and the biofilm formation of gentamicin-resistant strains. Among them, KLA (10 μg/mL) showed better antibacterial activity than the other three antimicrobial peptides against gentamicin-resistant K. pneumoniae.
As one of the important pathogens of community and nosocomial acquired infections, K. pneumoniae was ubiquitous in the natural environment. Due to the large and irregular use of antimicrobial agents, multiple drug-resistant strains appeared rapidly. The overall 14-day mortality rate of 249 patients infected with Klebsiella pneumoniae pneumonia in the intensive care unit was 28.9%.52 To determine drug-resistant K. pneumoniae and the resistance mechanisms, we compared the MIC value between strains screened by stepwise increasing concentrations of gentamicin and untreated strains. In our study, we found that five strains showed gentamicin resistance, especially the strain C and I.
The existence of the active efflux pump accounted for the multiple drug resistance of K. pneumoniae. Since the substrates of the active efflux system are not specific and very broad, some strains may constitute multiple types of different efflux systems simultaneously. When the efflux of these systems is enhanced, various antibacterial drugs are excreted through this system, resulting in K. pneumoniae resistance to multiple antibiotics, that is, multiple-drug resistance.53 The active efflux system consists of efflux proteins, fusion proteins and outer membrane channel proteins. Studies have shown that as the most important efflux system, AcrAB-TolC is composed of AcrA, AcrB and TolC,54 and widely exists in K. pneumoniae, accounting for the multiple drug resistance of K. pneumoniae.55 In addition, the porin genes (OmpK35 and OmpK36) and pump regulators (acrR) are closely related to drug resistance of K. pneumoniae.56 Our data showed that the mRNA levels of efflux pump genes (acrA, acrB, and tolC), the porin genes (OmpK35 and OmpK36) and pump regulators (acrR) were significantly increased in RT strains compared with WT strains. In addition, to further demonstrate whether the resistance of K. pneumoniae was attributable to the enhancement of efflux pump activity, CCCP was used to stimulate the RT strains and WT strains. As an efflux pump inhibitor, CCCP destroyed the proton gradient across the proteoliposome membrane of bacteria,57 resulting in the loss of energy of transporters and the increase in drug accumulation concentration in bacteria, thereby inhibiting drug efflux. In our study, EtBr accumulation assay demonstrated that the fluorescence value of EtBr in RT strains and WT strains was remarkably increased in the presence of CCCP, and the fluorescence value of RT strains was lower than that of WT strains in the presence of CCCP, suggesting that the drug resistance of K. pneumoniae was mediated by efflux pump activity, and the RT strains had stronger tolerance to drugs compared with the WT strains. The formation of biofilm is also one of the mechanisms of drug resistance.58 Biofilms block the infiltration of antimicrobial agents, making the antibacterial drugs ineffective. It has been reported that biofilm formation genes (mrkA, luxS, pga, wbbM, and wzm) are involved in K. pneumoniae biofilm.42 In this study, we found that the expression of biofilm formation genes (mrkA, luxS, pga, wbbM, and wzm) in strain C and I was significantly increased. The inhibition of gentamicin on the biofilm formation of RT strain C and I was weaker than that of WT strain.
In this study, we did not sequence the RT strains to detect DNA mutations. However, we found significant differences in the expression of genes related to efflux activity and biofilm formation between the RT strains and the WT strains. Future studies need to further analyze the genetic changes of RT strains and whether there are mutations in genes related to efflux activity and biofilm formation. To improve the conclusion of this paper.
Antibacterial peptides are an essential part of the natural immune system, characterized by high antibacterial efficiency and broad-spectrum. Unlike antibiotics, antimicrobial peptides rarely cause drug resistance to pathogens. Moreover, antimicrobial peptides have a certain killing effect on fungi, viruses, protozoa and tumor cells. Thus, the great potential of antimicrobial peptides is indicated for new drug development.59–63 According to statistics, 3099 natural antimicrobial peptides have been identified and isolated in the antimicrobial peptide database (as of May 2020, http://aps.unmc.edu/ap/main.php). In our study, four antimicrobial peptides (LL37, Magainin I, KLA and Dermaseptin) used to treat gentamicin-resistant K. pneumoniae infection significantly inhibited both biofilm formation and efflux activity of the RT strain (vs Gentamicin, P K. pneumoniae-infected lung cells and K. pneumoniae-induced pneumonia in mice. KLA significantly reduced the fluorescence intensity and annexin V-positive cell density of K. pneumoniae-infected lung cells (P K. pneumoniae infection. However, this study only compared the effects of gentamicin and four antimicrobial peptides on the treatment of gentamicin-resistant K. pneumoniae infection, and the lack of comparison with other antibiotics in the same class makes it difficult to determine the potential of KLA in the treatment of gentamicin-resistant K. pneumoniae infection. Therefore, other antibiotics and antimicrobial peptides will be added in the future to determine the advantages of antimicrobial peptides in the treatment of gentamicin-resistant K. pneumoniae infection. In addition, there is a lack of comparison of the differences in the effects of antimicrobial peptides on RT strains and WT strains in this study, which needs to be further studied in combination with the genetic changes of RT strains.
For all we know, K. pneumoniae is a pathogen of clinical relevance and can induce the inflammatory response of lung,64 while in vivo experiments revealed that KLA significantly reduced the bacterial load and levels of inflammatory cytokine in the lungs of mice with pneumonia and reduced the degree of lesions and the accumulation of neutrophils in the lung tissues, indicating that KLA could reduce inflammatory response to protect lung cells by destroying K. pneumoniae. It has been pointed out that the in vitro activity of antimicrobial peptides usually cannot be translated into in vivo efficacy due to the interference of the host microenvironment on peptide stability/efficacy.65 However, a recent studies have shown that neutrophil membrane coated nanoparticles mediated KLA peptides can evade immune system clearance and achieve efficient targeted aggregation and drug delivery at bacterial infection sites, effectively inhibiting the development of drug-resistant K. pneumonia pneumonia.34 Therefore, this study needs to be in for a particular infection in parts of the body model system to assess the effectiveness of antibacterial peptides, with more realistic to predict their successful treatment in clinical trials, and can be combined with the new body delivery method to maximize the effectiveness of antimicrobial peptides.
In addition, it has been shown that the activation of Toll and IMD pathways can induce the gene expression of various antimicrobial peptides,66 and NF-κB is also involved in the regulation,67 and Toll, IMD and NF-κB pathways are involved in oxidative stress68 and immune inflammation.69–71 In conclusion, antimicrobial peptides can significantly reduce the levels of inflammatory cytokines, which may be achieved through the Toll, IMD and NF-κB signaling pathway, that is, antimicrobial peptides may regulate inflammatory cytokines through the Toll, IMD and NF-κB signaling pathway to protect the lung tissue infected with gentamicin-resistant K. pneumonia. In the future, we will further explore the potential mechanisms of the four antimicrobial peptides destroying K. pneumonia, and supplement the downstream targets or signaling pathways of the four antimicrobial peptides regulating gentamicin-resistant K. pneumonia, so as to provide new ideas for the treatment of drug-resistant bacteria.
Conclusion
In Conclusion, the study revealed the effects of several antimicrobial peptides on gentamicin-resistant K. pneumoniae. Our data showed that four antimicrobial peptides exhibited antimicrobial activity against gentamicin-resistant K. pneumoniae strains, especially KLA, indicating that KLA was a promising antimicrobial peptide candidate for treating infections caused by K. pneumoniae.
Data Sharing Statement
Datasets analyzed during the current study are available from the corresponding author on reasonable request.
Ethics Approval and Consent to Participate
All experiments were conducted with the informed consent of the subjects, and the experimental protocol complied with the Declaration of Helsinki was approved by the Medical Ethics Committee of Wuxi Fifth People’s Hospital (2021-021-1). All animal experiments complied with the welfare of laboratory Animals and the ethical requirements of animal testing (Laboratory animal—Guideline for ethical review of animal welfare, GB/T 35892-2018). The animal experiment protocol was approved by the Animal Ethics Committee of Jiangnan University (20220302-WY001). All experiments were conducted with the informed consent of the subjects.
Funding
This work was supported by the Major Projects of Wuxi Municipal Health Commission (No. Z202111).
Disclosure
The authors report no conflicts of interest in this work.
References
1. Bassetti M, Righi E, Carnelutti A, Graziano E, Russo A. Multidrug-resistant Klebsiella pneumoniae: challenges for treatment, prevention and infection control. Expert Rev Anti Infect Ther. 2018;16:749–761. doi:10.1080/14787210.2018.1522249
2. Anes J, Hurley D, Martins M, Fanning S. Exploring the genome and phenotype of multi-drug resistant Klebsiella pneumoniae of clinical origin. Front Microbiol. 2017;8:1913. doi:10.3389/fmicb.2017.01913
3. Heinz E, Ejaz H, Bartholdson Scott J, et al. Resistance mechanisms and population structure of highly drug resistant Klebsiella in Pakistan during the Introduction of the carbapenemase NDM-1. Sci Rep. 2019;9:2392. doi:10.1038/s41598-019-38943-7
4. Lin WH, Kao CY, Yang DC, et al. Clinical and microbiological characteristics of Klebsiella pneumoniae from community-acquired recurrent urinary tract infections. Eur J Clin Microbiol Infect Dis. 2014;33:1533–1539. doi:10.1007/s10096-014-2100-4
5. Barbadoro P, Labricciosa FM, Recanatini C, et al. Catheter-associated urinary tract infection: role of the setting of catheter insertion. Am J Infect Control. 2015;43:707–710. doi:10.1016/j.ajic.2015.02.011
6. Gu D, Dong N, Zheng Z, et al. A fatal outbreak of ST11 carbapenem-resistant hypervirulent Klebsiella pneumoniae in a Chinese hospital: a molecular epidemiological study. Lancet. 2018;18:37–46. doi:10.1016/S1473-3099(17)30489-9
7. Ikuta KS, Sharara F; Antimicrobial Resistance, C. Global burden of bacterial antimicrobial resistance in 2019: a systematic analysis. Lancet. 2022;399:629–655. doi:10.1016/S0140-6736(21)02724-0
8. Choby JE, Howard-Anderson J, Weiss DS. Hypervirulent Klebsiella pneumoniae – clinical and molecular perspectives. J Intern Med. 2020;287:283–300. doi:10.1111/joim.13007
9. Effah CY, Sun T, Liu S, Wu Y. Klebsiella pneumoniae: an increasing threat to public health. Ann Clin Microbiol Antimicrob. 2020;19:1. doi:10.1186/s12941-019-0343-8
10. Wang G, Zhao G, Chao X, Xie L, Wang H. The characteristic of virulence, biofilm and antibiotic resistance of Klebsiella pneumoniae. Int J Environ Res Public Health. 2020;17. doi:10.3390/ijerph17176278
11. Esposito S, De Simone G. Update on the main MDR pathogens: prevalence and treatment options. Infez Med. 2017;25:301–310.
12. Falagas ME, Karageorgopoulos DE. Extended-spectrum beta-lactamase-producing organisms. J Hosp Infect. 2009;73:345–354. doi:10.1016/j.jhin.2009.02.021
13. Rodriguez-Martinez JM, Cano ME, Velasco C, Martinez-Martinez L, Pascual A. Plasmid-mediated quinolone resistance: an update. J Infect Chemother. 2011;17:149–182. doi:10.1007/s10156-010-0120-2
14. Nikaido H. Preventing drug access to targets: cell surface permeability barriers and active efflux in bacteria. Semin Cell Dev Biol. 2001;12:215–223. doi:10.1006/scdb.2000.0247
15. Hernando-Amado S, Blanco P, Alcalde-Rico M, et al. Multidrug efflux pumps as main players in intrinsic and acquired resistance to antimicrobials. Drug Resist Updat. 2016;28:13–27. doi:10.1016/j.drup.2016.06.007
16. Li XZ, Plesiat P, Nikaido H. The challenge of efflux-mediated antibiotic resistance in Gram-negative bacteria. Clin Microbiol Rev. 2015;28:337–418. doi:10.1128/CMR.00117-14
17. Alav I, Sutton JM, Rahman KM. Role of bacterial efflux pumps in biofilm formation. J Antimicrob Chemother. 2018;73:2003–2020. doi:10.1093/jac/dky042
18. Gemeinder JLP, Barros NRD, Pegorin GS, et al. Gentamicin encapsulated within a biopolymer for the treatment of Staphylococcus aureus and Escherichia coli infected skin ulcers. J Biomater Sci Polym Ed. 2021;32:93–111. doi:10.1080/09205063.2020.1817667
19. Avent ML, Rogers BA, Cheng AC, Paterson DL. Current use of aminoglycosides: indications, pharmacokinetics and monitoring for toxicity. Intern Med J. 2011;41:441–449. doi:10.1111/j.1445-5994.2011.02452.x
20. Appel GB, Neu HC. Gentamicin in 1978. Ann Intern Med. 1978;89:528–538. doi:10.7326/0003-4819-89-4-528
21. Duffy CR, Huang Y, Andrikopoulou M, et al. Clindamycin, gentamicin, and risk of clostridium difficile infection and acute kidney injury during delivery hospitalizations. Obstet Gynecol. 2020;135:59–67. doi:10.1097/AOG.0000000000003568
22. Li X, Hu Q, Lin Q, et al. Inhibition of Candida albicans in vivo and in vitro by antimicrobial peptides chromogranin A-N12 through microRNA-155/suppressor of cytokine signaling 1 axis. Bioengineered. 2022;13:2513–2524. doi:10.1080/21655979.2021.2017680
23. Lazzaro BP, Zasloff M, Rolff J. Antimicrobial peptides: application informed by evolution. Science. 2020;368. doi:10.1126/science.aau5480
24. Mwangi J, Hao X, Lai R, Zhang ZY. Antimicrobial peptides: new hope in the war against multidrug resistance. Zool Res. 2019;40:488–505. doi:10.24272/j.issn.2095-8137.2019.062
25. da Costa JP, Cova M, Ferreira R, Vitorino R. Antimicrobial peptides: an alternative for innovative medicines? Appl Microbiol Biotechnol. 2015;99:2023–2040. doi:10.1007/s00253-015-6375-x
26. Lai Y, Gallo RL. AMPed up immunity: how antimicrobial peptides have multiple roles in immune defense. Trends Immunol. 2009;30:131–141. doi:10.1016/j.it.2008.12.003
27. Mahlapuu M, Hakansson J, Ringstad L, Bjorn C. Antimicrobial peptides: an emerging category of therapeutic agents. Front Cell Infect Microbiol. 2016;6:194. doi:10.3389/fcimb.2016.00194
28. Nagaoka I, Tamura H, Reich J. Therapeutic potential of cathelicidin peptide LL-37, an antimicrobial agent, in a murine sepsis model. Int J Mol Sci. 2020;21:5973. doi:10.3390/ijms21175973
29. Memariani H, Memariani M. Antibiofilm properties of cathelicidin LL-37: an in-depth review. World J Microbiol Biotechnol. 2023;39:99. doi:10.1007/s11274-023-03545-z
30. Denardi LB, de Arruda Trindade P, Weiblen C, et al. In vitro activity of the antimicrobial peptides h-Lf1-11, MSI-78, LL-37, fengycin 2B, and magainin-2 against clinically important bacteria. Braz J Microbiol. 2022;53:171–177. doi:10.1007/s42770-021-00645-6
31. Cardoso MH, de Almeida KC, Cândido ES, et al. Comparative transcriptome analyses of magainin I-susceptible and -resistant Escherichia coli strains. Microbiology (Reading). 2018;164:1383–1393. doi:10.1099/mic.0.000725
32. Hacioglu M, Oyardi O, Bozkurt-Guzel C, Savage PB. Antibiofilm activities of ceragenins and antimicrobial peptides against fungal-bacterial mono and multispecies biofilms. J Antibiot (Tokyo). 2020;73:455–462. doi:10.1038/s41429-020-0299-0
33. Hu C, Chen X, Huang Y, Chen Y. Synergistic effect of the pro-apoptosis peptide kla-TAT and the cationic anticancer peptide HPRP-A1. Apoptosis. 2018;23:132–142. doi:10.1007/s10495-018-1443-1
34. Liu J, Chen X, Xu L, et al. Neutrophil membrane-coated nanoparticles exhibit increased antimicrobial activities in an anti-microbial resistant K. pneumonia infection model. Nanomedicine. 2023;48:102640. doi:10.1016/j.nano.2022.102640
35. Zai Y, Ying Y, Ye Z, et al. Broad-spectrum antimicrobial activity and improved stability of a D-amino acid enantiomer of DMPC-10A, the designed derivative of dermaseptin truncates. Antibiotics. 2020;9. doi:10.3390/antibiotics9090627
36. Dong Z, Zhang H, Gong X, et al. The role of the tumor microenvironment in neuropilin 1-induced radiation resistance in lung cancer cells. J Cancer. 2019;10:4017–4030. doi:10.7150/jca.28163
37. Fisher ML, Grun D, Adhikary G, Xu W, Eckert RL. Inhibition of YAP function overcomes BRAF inhibitor resistance in melanoma cancer stem cells. Oncotarget. 2017;8:110257–110272. doi:10.18632/oncotarget.22628
38. Sun ZW, Zhang L, Zhu SJ, Chen WC, Mei B. Excitotoxicity effects of glutamate on human neuroblastoma SH-SY5Y cells via oxidative damage. Neurosci Bull. 2010;26:8–16. doi:10.1007/s12264-010-0813-7
39. Singulani JL, Oliveira LT, Ramos MD, et al. The antimicrobial peptide MK58911-NH 2 acts on planktonic, biofilm, and intramacrophage cells of Cryptococcus neoformans. Antimicrob. Agents Chemother. 2021;65:e0090421. doi:10.1128/aac.00904-21
40. Gorr SU, Flory CM, Schumacher RJ. In vivo activity and low toxicity of the second-generation antimicrobial peptide DGL13K. PLoS One. 2019;14:e0216669. doi:10.1371/journal.pone.0216669
41. Padilla E, Llobet E, Domenech-Sanchez A, et al. Klebsiella pneumoniae AcrAB efflux pump contributes to antimicrobial resistance and virulence. Antimicrob Agents Chemother. 2010;54:177–183. doi:10.1128/AAC.00715-09
42. Vuotto C, Longo F, Pascolini C, et al. Biofilm formation and antibiotic resistance in Klebsiella pneumoniae urinary strains. J Appl Microbiol. 2017;123:1003–1018. doi:10.1111/jam.13533
43. Livak KJ, Schmittgen TD. Analysis of relative gene expression data using real-time quantitative PCR and the 2(-Delta Delta C(T)) Method. Methods. 2001;25:402–408. doi:10.1006/meth.2001.1262
44. Yousefpour Z, Davarzani F, Owlia P. Evaluating of the effects of Sub-MIC concentrations of gentamicin on biofilm formation in clinical isolates of pseudomonas aeruginosa. Iran j Pathol. 2021;16:403–410. doi:10.30699/ijp.20201.524220.2584
45. Lin Q, Deslouches B, Montelaro RC, Di YP. Prevention of ESKAPE pathogen biofilm formation by antimicrobial peptides WLBU2 and LL37. Int J Antimicrob Agents. 2018;52:667–672. doi:10.1016/j.ijantimicag.2018.04.019
46. Ahmed D, Anwar A, Khan AK, et al. Size selectivity in antibiofilm activity of 3-(diphenylphosphino)propanoic acid coated gold nanomaterials against Gram-positive Staphylococcus aureus and Streptococcus mutans. AMB Express. 2017;7:210. doi:10.1186/s13568-017-0515-x
47. Wu T, Li H, Su C, et al. Microbiota-derived short-chain fatty acids promote LAMTOR2-mediated immune responses in macrophages. mSystems. 2020;5. doi:10.1128/mSystems.00587-20
48. Zheng ZQ, Li Z-X, Zhou G-Q, et al. Long Noncoding RNA fam225a promotes nasopharyngeal carcinoma tumorigenesis and metastasis by acting as ceRNA to sponge miR-590-3p/miR-1275 and upregulate ITGB3. Cancer Res. 2019;79:4612–4626. doi:10.1158/0008-5472.CAN-19-0799
49. Zhao S, Tang L, Chen W, et al. Resveratrol-induced apoptosis is associated with regulating the miR-492/CD147 pathway in malignant melanoma cells. Naunyn Schmiedebergs Arch Pharmacol. 2021;394:797–807. doi:10.1007/s00210-020-01981-4
50. Aujla SJ, Chan YR, Zheng M, et al. IL-22 mediates mucosal host defense against Gram-negative bacterial pneumonia. Nat Med. 2008;14:275–281. doi:10.1038/nm1710
51. Bansal S, Harjai K, Chhibber S. Depolymerase improves gentamicin efficacy during Klebsiella pneumoniae induced murine infection. BMC Infect Dis. 2014;14:456. doi:10.1186/1471-2334-14-456
52. Tran TN, Vu DH, Nguyen HA, et al. Predicting mortality in intensive care unit patients infected with Klebsiella pneumoniae: a retrospective cohort study. J Infect Chemother. 2022;28:10–18. doi:10.1016/j.jiac.2021.09.001
53. Piddock LJV. The 2019 Garrod Lecture: MDR efflux in Gram-negative bacteria-how understanding resistance led to a new tool for drug discovery. J Antimicrob Chemother. 2019;74:3128–3134. doi:10.1093/jac/dkz370
54. Nazarov PA, Kotova EA, Skulachev VP, Antonenko YN. Genetic variability of the AcrAB-TolC multidrug efflux pump underlies SkQ1 resistance in gram-negative bacteria. Acta Naturae. 2019;11:93–98. doi:10.32607/20758251-2019-11-4-93-98
55. Bialek-Davenet S, Lavigne J-P, Guyot K, et al. Differential contribution of AcrAB and OqxAB efflux pumps to multidrug resistance and virulence in Klebsiella pneumoniae. J Antimicrob Chemother. 2015;70:81–88. doi:10.1093/jac/dku340
56. Hamzaoui Z, Ocampo-Sosa A, Maamar E, et al. An outbreak of NDM-1-producing Klebsiella pneumoniae, associated with OmpK35 and OmpK36 porin loss in Tunisia. Microb Drug Resist. 2018;24:1137–1147. doi:10.1089/mdr.2017.0165
57. Nuber F, Mérono L, Oppermann S, et al. A quinol anion as catalytic intermediate coupling proton translocation with electron transfer in E. coli respiratory complex I. Front Chem. 2021;9:672969. doi:10.3389/fchem.2021.672969
58. Dai W, Zhang Y, Zhang J, et al. Analysis of antibiotic-induced drug resistance of Salmonella enteritidis and its biofilm formation mechanism. Bioengineered. 2021;12:10254–10263. doi:10.1080/21655979.2021.1988251
59. Mulani MS, Kamble EE, Kumkar SN, Tawre MS, Pardesi KR. Emerging strategies to combat ESKAPE pathogens in the era of antimicrobial resistance: a review. Front Microbiol. 2019;10:539. doi:10.3389/fmicb.2019.00539
60. Divyashree M, Mani MK, Reddy D, et al. Clinical applications of antimicrobial peptides (AMPs): where do we stand now? Protein Pept Lett. 2020;27:120–134. doi:10.2174/0929866526666190925152957
61. Lei J, Sun L, Huang S, et al. The antimicrobial peptides and their potential clinical applications. Am J Transl Res. 2019;11:3919–3931.
62. de Freire Bastos MDC, Miceli de Farias F, Carlin Fagundes P, Varella Coelho ML. Staphylococcins: an update on antimicrobial peptides produced by staphylococci and their diverse potential applications. Appl Microbiol Biotechnol. 2020;104:10339–10368. doi:10.1007/s00253-020-10946-9
63. Rollins-Smith LA. The role of amphibian antimicrobial peptides in protection of amphibians from pathogens linked to global amphibian declines. Biochim Biophys Acta. 2009;1788:1593–1599. doi:10.1016/j.bbamem.2009.03.008
64. Kumar V, Harjai K, Chhibber S. 2-Chloroadenosine (2-CADO) treatment modulates the pro-inflammatory immune response to prevent acute lung inflammation in BALB/c mice suffering from Klebsiella pneumoniae B5055-induced pneumonia. Int J Antimicrob Agents. 2010;35:599–602. doi:10.1016/j.ijantimicag.2010.01.014
65. Grassi L, Batoni G, Ostyn L, et al. The antimicrobial peptide lin-SB056-1 and its dendrimeric derivative prevent pseudomonas aeruginosa biofilm formation in physiologically relevant models of chronic infections. Front Microbiol. 2019;10:198. doi:10.3389/fmicb.2019.00198
66. Shrestha S, Kim Y. Activation of immune-associated phospholipase A2 is functionally linked to Toll/Imd signal pathways in the red flour beetle, Tribolium castaneum. Dev Comp Immunol. 2010;34:530–537. doi:10.1016/j.dci.2009.12.013
67. Tang R, Huang W, Guan J, et al. Drosophila H2Av negatively regulates the activity of the IMD pathway via facilitating Relish SUMOylation. PLoS Genet. 2021;17:e1009718. doi:10.1371/journal.pgen.1009718
68. Sun C, Shan F, Liu M, et al. High-fat-diet-induced oxidative stress in giant freshwater prawn (Macrobrachium rosenbergii) via NF-kappaB/NO signal pathway and the amelioration of vitamin E. Antioxidants. 2022;11. doi:10.3390/antiox11020228
69. Sajjadian SM, Vatanparast M, Kim Y. Toll/IMD signal pathways mediate cellular immune responses via induction of intracellular PLA (2) expression. Arch Insect Biochem Physiol. 2019;101:e21559. doi:10.1002/arch.21559
70. Bangi E. Drosophila at the intersection of infection, inflammation, and cancer. Front Cell Infect Microbiol. 2013;3:103. doi:10.3389/fcimb.2013.00103
71. Tendulkar S, Hegde S, Garg L, et al. Caspar, an adapter for VAPB and TER94, modulates the progression of ALS8 by regulating IMD/NFκB-mediated glial inflammation in a Drosophila model of human disease. Hum Mol Genet. 2022;31:2857–2875. doi:10.1093/hmg/ddac076