Tech
Association of EEG Power during Sleep and Attention Levels | NSS
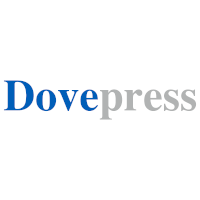
Introduction
Major depressive disorder (MDD) is a mental disorder with an estimated 322 million patients worldwide, accounting for 4.4% of the world’s population.1 It has been reported that patients with MDD have cognitive impairment, as reflected by poor attention, executive function, visual learning, and memory.2 However, the underlying mechanisms are still not clear.2–4
Impaired sleep is common in MDD, affecting 50–90% of patients with MDD.5,6 Studies showed that patients with MDD have disinhibition of rapid eye movement (REM) sleep (ie, shortening REM sleep latency and increased REM density),7 which is a specific marker for MDD. In addition, MDD also manifests as impaired sleep quality (ie, prolonged sleep latency, increased intermittent awakenings, early morning awakenings, and more NREM stage 1 sleep).8 Furthermore, it has been shown that patients with MDD, during sleep, have lower levels of delta and theta power9,10 and greater beta activity.11
Several studies have suggested that EEG activity during sleep is associated with cognitive function.12,13 However, the findings are inconsistent. For example, a study reported that less delta power during NREM sleep was associated with worse cognitive function,13 while another study found that greater delta power during REM sleep was associated with slower reaction times.14 The inconsistent findings might be associated these studies included different subjects.
Taken together, though both cognitive dysfunction and sleep impairment are common features of MDD, no studies have examined the association between sleep and cognition in patients with MDD. Thus, the aim of this study was to examine the association between EEG activity during sleep and attention levels in patients with MDD.
Materials and Methods
Participants
This was a between-group and cross-sectional design study conducted at the Sleep Medicine Center of Shantou University Medical College. Patients with MDD and non-MDD control subjects matched for age and gender comprised the study sample. This study was approved by the Research Ethics Board of the Mental Health Center of Shantou University and informed consent was obtained from each participant. This study complied with the Declaration of Helsinki.
Consecutive MDD outpatients (age ≥18 years) were recruited from the clinic of the Mental Health Center of Shantou University. Control subjects were consecutively recruited through advertisements on the college campus and the community during the same period. A complete medical history and mental status assessment was performed. All potential research subjects were interviewed with a comprehensive questionnaire. The questionnaire gathered a history of sleep complaints, general health issues, and medication use. Inclusion criteria for the MDD group included (1) currently met the diagnostic criteria for MDD according to the Diagnostic and Statistical Manual of Mental Disorders (DSM-5) criteria, whether or not the first onset of MDD; (2) not under any psychotropic medication treatment currently or in past one month and (3) right-handed. We excluded patients with MDD who had (1) other psychiatric disorders, such as bipolar disorder, schizophrenia, or psychotic developmental disorder, (2) a diagnosis of dementia or other advanced cognitive impairment, (3) evidence of sleep apnea, based on a polysomnographic (PSG)-measured apnea–hypopnea index (AHI) ≥ 5 events per hour, (4) extreme sleep schedules and/or were shift workers, or were (5) pregnant/nursing. Controls were adults who were not suffering from any psychiatric disorder currently or in the past and were right-handed. Exclusion criteria for control subjects were the same as that for patients with MDD.
A sample size calculation was performed based on a previous report,2 the mean difference between the attention of patients with MDD and controls was 0.36. The sample size was calculated using PASS 15.0 software, and the significance level α was set to 0.05, β was 0.1, statistical power was 0.9, and σ was 0.5. In the ratio of 1:1, at least 42 patients with MDD and 42 people in the control group were required. Consequently, in this study, we included 47 patients with MDD and 47 control subjects.
Measures
All participants completed a medical history and physical examination using a semi-structured questionnaire and a series of clinical examinations. The Pittsburgh sleep quality index (PSQI) and the Epworth sleepiness scale (ESS) were to assess subjective sleep quality15 and daytime sleepiness,16 respectively. Anthropometric Measures included height and weight measurements according to standard procedures in the sleep laboratory. Body mass index (BMI) was calculated based on weight (kg)/height (m2).
Mood Status
The Beck Depression Inventory (BDI)17 and Beck Anxiety Inventory (BAI)18 were used to assess depression and anxiety symptoms. A higher total score indicates more severe symptoms of depression and anxiety.
Polysomnography
All participants underwent an overnight-attended PSG examination in the sleep laboratory. During the PSG evaluation, subjects were allowed to sleep based on their habitual sleep time, with the recording time ranging from 22:00–23:00 hours to 6:00–7:00 hours. Participants were continuously monitored with 16-channel polygraphs, including electroencephalogram, bilateral electrooculogram, electromyogram, and electrocardiogram. All sleep parameters recorded by PSG were analyzed and scored according to the international criteria of the American Academy of Sleep Medicine19 by a senior technician who was blind to any diagnosis.
Spectral Analyses
Spectral analyses of EEG data were performed on central EEG derivations (C3-M2 and C4-M1) during NREM and REM sleep, as in our previous study,20 using the fast Fourier transform (FFT) to estimate power spectra.21 After automatically excluded epochs containing movements, arousal and poor contact of electrodes, artifact-free EEG data from NREM sleep and REM sleep were selected. For spectral analyses, NREM sleep was defined as any period of NREM sleep lasting at least 15 minutes, and REM sleep was defined as any period of REM sleep lasting at least 5 minutes. The spectral resolution was set to 0.50 Hz in each epoch. Following the application of a Hann window, spectral power was calculated within each FFT window.20,22 All artifact-free epochs of NREM sleep and REM sleep were used to obtain overnight spectral power. To calculate relative power, the absolute power of each frequency band was divided by the total power of all frequency bands. This study used the averaged C3-M2 and C4-M1 relative power to perform analyses.23 The ranges corresponding to the different relative powers calculated for each epoch were as follows: delta was defined as 0.5–4.0 Hz, theta as 4.5–8.0 Hz, alpha as 8.5–11.0 Hz, sigma as 11.5–15.0 Hz, beta as 15.5–30.0 Hz and gamma as 30.5 −49.5 Hz.
Attention Network Test
The attention network test (ANT), which is designed to evaluate alerting, orienting, and executive control attention within a single 30-min testing session,24 was performed using E-prime software25 in the morning (09:30–10:30 h) following PSG recording in the sleep laboratory. Subjects were required to focus their attention on a centrally located fixation cross during the test session, and to respond as quickly and accurately as possible by pressing the appropriate button on the keyboard according to the target arrow direction. The scores for alerting, orienting, and executive control attention were obtained by subtraction of reaction time (RT) under various conditions.25 The higher the values of the alerting and orienting effects, the better the alerting and orienting attention performance, respectively. However, the lower the value of the executive control effect, the better the executive control attention performance.
Statistical Analysis
Data are presented as the mean ± standard deviation (SD) for continuous variables, and frequency and percent for categorical variables. The independent t-test and Mann–Whitney U-test were used for normally and non-normally distributed continuous variables, respectively, and the χ2 test was used for categorical variables to compare general demographic characteristics, clinical characteristics, and sleep characteristics between groups. Analysis of covariance (ANCOVA) was used to analyze the differences in EEG power between patients with MDD and control subjects, with age and gender as covariates. Considering age26 and gender26 may affect EEG power during sleep, we adjusted these variables in ANCOVA. Differences in attention levels between patients with MDD and controls were analyzed using ANCOVA. In addition to age and gender, considering BMI27 and education levels28 may affect cognitive function, we also adjusted for BMI and education levels for the comparisons regarding attention levels. ANCOVA results are reported as mean ± standard error of the mean (SEM). Furthermore, we used linear regression models to examine the association between EEG power during sleep and attention levels with adjustment of age, gender, BMI, and education levels in patients with MDD and controls, respectively. We selected the EEG power variables and the cognition-related variables that were significantly different between patients with MDD and controls as independent variables and dependent variables, respectively. Then, each selected EEG variable and cognition variable will be treated as an independent variable and a dependent variable with adjustment of confounding factors in a linear regression model. Regarding effect size, we calculated Partial eta squared (η2p) and standardized beta value (β) for the ANCOVA and linear regression analysis, respectively. A P-value ≤0.05 was used to determine statistical significance. All analyses were conducted using SPSS 25.0 (IBM Corp).
Results
Forty-seven patients with MDD and forty-seven age-sex-matched controls were included in this study. Demographic, clinical, and sleep characteristics for patients with MDD and control subjects are presented in Table 1. Patients with MDD had lower education levels, shorter total sleep time, lower sleep efficiency, and longer wake time after sleep onset latency compared to control subjects (Table 1).
Table 1 Demographic, Clinical and Sleep Characteristics |
EEG Spectral Analyses
As shown in Table 2, after controlling for age and gender, compared to controls, patients with MDD had lower theta power during NREM (10.06 ± 0.29% vs 11.06 ± 0.29%, P = 0.018) and REM (16.02 ± 0.43% vs 17.97 ± 0.43%, P = 0.002) sleep. Furthermore, we found that patients with MDD had higher beta power (2.22 ± 0.12% vs 1.90 ± 0.12%, P = 0.050) during NREM sleep and delta power (67.70 ± 0.87% vs 64.73 ± 0.87%, P = 0.018) during REM sleep. In addition, there were no statistical differences in other EEG power bands between the two groups.
![]() |
Table 2 EEG Power of Controls and Patients with MDD |
Attention Performance
As shown in Table 3, compared with controls, patients with MDD had lower accuracy (93.95 ± 1.02% vs 97.41 ± 1.02%, P = 0.021), longer mean RT (646.79 ± 15.56 ms vs 569.18 ± 15.56 ms, P P = 0.038) and worse executive control (108.01 ms ± 7.16 vs 87.33 ± 7.16 ms, P = 0.048), after controlling for age, gender, BMI and education levels. There were no differences regarding orienting (P = 0.113) between patients with MDD and controls.
![]() |
Table 3 Attention Levels of Controls and Patients with MDD |
Association Between EEG Spectra and Attention
In order to examine the association between EEG power during sleep and attention levels in MDD, we conducted linear regression in patients with MDD. As shown in Table 4, decreased theta power during NREM sleep was associated with worsened accuracy (β = 0.329, P = 0.040), and decreased theta power during REM sleep was associated with worsened alerting effect (β = 0.355, P = 0.020), and increased delta power during REM sleep was associated with prolonged mean RT (β = 0.325, P = 0.022), in patients with MDD after controlling for age, gender, BMI, and educational levels. No association between ANT performance and other frequency bands was observed in patients with MDD. However, higher beta power during NREM sleep was associated with better executive control in controls was observed (β = −0.316, P = 0.026).
![]() |
Table 4 Association Between EEG Power and ANT Index in Patients with MDD and Control Subjects |
Discussion
To our knowledge, this is the first study to examine the relationship between EEG power during sleep and attention levels in patients with MDD. Our findings suggest that decreased theta power during NREM sleep and increased delta and decreased theta power during REM sleep are associated with impaired attention levels in patients with MDD.
In the current study, we found that decreased relative theta EEG power occurs in patients with MDD both during NREM sleep and REM sleep, which is consistent with previous studies.10,29 The decreased levels of theta power during sleep have been shown to be associated with impaired cognition (ie, memory consolidation).30,31 Elevated beta activity during NREM sleep has been reported in depressive patients.11 Consistent with previous studies, we found that the relative beta power during NREM sleep in patients with MDD is higher than controls. Higher levels of beta activity during sleep are currently thought to be involved in behavioral arousal and are accompanied by increased cortical arousal events.32,33 Furthermore, increased levels of delta power during REM sleep were also observed in patients with MDD in the current study. A previous study reported that patients with MDD had lower delta power during NREM sleep.9 Although this study found that patients with MDD had lower integrated EEG power density compared to controls, the authors did not report delta power during REM sleep in patients with MDD.9 However, findings from many studies show that increased levels of delta activity during REM sleep are associated with poorer sleep quality both in healthy individuals and in a variety of diseases.34–36 The increased levels of delta activity during REM sleep in MDD might be associated with poor sleep quality. In our study, we found that patients with MDD had higher PSQI scores, lower sleep efficiency and longer wake time after sleep onset compared to control subjects.
Attention impairment is one of the common complaints in patients with MDD. In the current study, we found that patients with MDD had impaired attention in multiple dimensions (ie, lower accuracy, longer reaction time, worse alerting levels, and poorer executive control) compared with controls, which is consistent with most, but not all previous studies.37–45 The inconsistent findings might be due to the effects of medication (eg, antidepressants) and potential confounding factors that some studies did not take into consideration.
Theta EEG power is generated by the thalamus and is associated with inhibition of alertness.46 Animal studies suggested that theta EEG power generates from hippocampus, which is a region closely associated with cognitive function.47,48 Evidence from human studies also reported that decreased theta EEG power was associated with worse cognitive performance, such as attention and memory.49 In the current study, lower levels of theta activity during NREM sleep are associated with lower accuracy in patients with MDD, which is in line with a previous finding that reduced levels of theta activity during NREM sleep are associated with early cognitive impairment.50 Furthermore, we found that lower levels of theta activity during REM sleep are associated with worse alertness levels in patients with MDD, which is inconsistent with a previous study showing that elevated theta activity during REM sleep is concomitant with worse cognitive performance.51
In addition, we found that greater levels of delta activity during REM sleep are associated with slower reaction time in patients with MDD. A previous study showed that increased delta power during REM sleep is associated with longer reaction time,14 which is consistent with our study. Furthermore, evidence has shown that greater delta activity during REM sleep is associated with impaired cognition.12,52 Findings of an animal study suggested that increased delta power during sleep was associated with activating the adenosine A1 receptor in the hippocampus,53 which has been observed to be associated with impaired cognitive function in aged mice.54 Furthermore, elevated delta power during REM sleep has been observed to be associated with poor cognitive performance in humans.55 Future studies should be conducted to understand the mechanisms underlying the association between sleep and cognition in patients with MDD.
In contrast to patients with MDD, we found that higher levels of beta power during NREM sleep were associated with better executive control in control subjects. Higher relative power in high-frequency EEG power NREM sleep has been reported to be associated with better cognitive performance,51 while lower levels of high-frequency EEG power during NREM sleep were observed in the subjects with cognitive impairment.56 Thus, it appears that elevated beta power during NREM sleep may contribute to better cognitive function in the non-MDD population.
The strengths in the present study include the careful consideration of potential influencing factors (eg, age, gender, medication and education) that may affect attention performance and EEG activity during sleep. However, several limitations also need to be acknowledged and merit discussed. First, it is a cross-sectional study that can not provide a causal relationship between EEG activity during sleep and attention levels in patients with MDD. Second, the gamma EEG power was filtered out during the acquisition process, so the relationship between gamma power and attention performance could not be evaluated. Third, we primarily included young and middle-aged individuals, which restricted the findings to children, adolescents and older patients with MDD. Future studies should be conducted in children, adolescents, and older adult depressive patients and expanded to other dimensions of cognitive function, such as memory, executive function, and information processing speed. Finally, we did not collect information regarding social jet lag, which may also affect cognitive function.
Conclusions
Our findings suggest that patients with MDD manifest impaired selective attention function that is associated with decreased theta EEG power during NREM/REM sleep and increased delta EEG power during REM sleep.
Abbreviations
AHI, apnea hypopnea index; ANCOVA, Analysis of covariance; BAI, Beck Anxiety Inventory; BDI, Beck Depression Inventory; BMI, body mass index; EEG, electroencephalogram; ESS, Epworth Sleepiness Scale; MDD, major depressive disorder; NREM, non-rapid eye movement; PSQI, Pittsburgh Sleep Quality Index; REM, rapid eye movement; RT, reaction time; SEM, standard error of the mean.
Data Sharing Statement
Data and protocols are available upon reasonable request. Data and protocols are available on request from the corresponding author.
Acknowledgments
Weiyu Cai and Le Chen are co-first authors for this study. The authors thank the sleep technicians and staff of the Department of Sleep Medicine, Shantou University Mental Health Center, for their support with this project.
Author Contributions
All authors made a significant contribution to the work reported, whether that is in the conception, study design, execution, acquisition of data, analysis and interpretation, or in all these areas; took part in drafting, revising or critically reviewing the article; gave final approval of the version to be published; have agreed on the journal to which the article has been submitted; and agree to be accountable for all aspects of the work.
Funding
This study was supported by the National Natural Science Foundation of China [No. 81970087; No. 82270105] and 2020 Li Ka Shing Foundation Cross-Disciplinary Research Grant [2020LKSFG05B]. These funding sources had no role in the design and conduct of the study; collection, management, analysis, and interpretation of the data; preparation, review, or approval of the manuscript; and decision to submit the manuscript for publication.
Disclosure
All authors report no potential conflicts of interest for this work.
References
1. Friedrich MJ. Depression is the leading cause of disability around the world. JAMA. 2017;317(15):1517. doi:10.1001/jama.2017.3826
2. Lee RS, Hermens DF, Porter MA, Redoblado-Hodge MA. A meta-analysis of cognitive deficits in first-episode Major Depressive Disorder. J Affect Disord. 2012;140(2):113–124. doi:10.1016/j.jad.2011.10.023
3. Nikolin S, Tan YY, Martin D, Moffa A, Loo CK, Boonstra TW. Behavioural and neurophysiological differences in working memory function of depressed patients and healthy controls. J Affect Disord. 2021;295:559–568. doi:10.1016/j.jad.2021.08.083
4. Hammar Å, Ronold EH, Rekkedal G. Cognitive impairment and neurocognitive profiles in major depression-a clinical perspective. Front Psychiatry. 2022;13:764374. doi:10.3389/fpsyt.2022.764374
5. Tsuno N, Besset A, Ritchie K. Sleep and depression. J Clin Psychiatry. 2005;66(10):1254–1269. doi:10.4088/jcp.v66n1008
6. Geoffroy PA, Hoertel N, Etain B, et al. Insomnia and hypersomnia in major depressive episode: prevalence, sociodemographic characteristics and psychiatric comorbidity in a population-based study. J Affect Disord. 2018;226:132–141. doi:10.1016/j.jad.2017.09.032
7. Arfken CL, Joseph A, Sandhu GR, Roehrs T, Douglass AB, Boutros NN. The status of sleep abnormalities as a diagnostic test for major depressive disorder. J Affect Disord. 2014;156:36–45. doi:10.1016/j.jad.2013.12.007
8. Ilanković A, Damjanović A, Ilanković V, Filipović B, Janković S, Ilanković N. Polysomnographic sleep patterns in depressive, schizophrenic and healthy subjects. Psychiatry Danub. 2014;26(1):20–26.
9. Borbély AA, Tobler I, Loepfe M, et al. All-night spectral analysis of the sleep EEG in untreated depressives and normal controls. Psychiatry Res. 1984;12(1):27–33. doi:10.1016/0165-1781(84)90135-5
10. Cheng P, Goldschmied J, Deldin P, Hoffmann R, Armitage R. The role of fast and slow EEG activity during sleep in males and females with major depressive disorder. Psychophysiology. 2015;52(10):1375–1381. doi:10.1111/psyp.12472
11. Nofzinger EA, Price JC, Meltzer CC, et al. Towards a neurobiology of dysfunctional arousal in depression: the relationship between beta EEG power and regional cerebral glucose metabolism during NREM sleep. Psychiatry Res. 2000;98(2):71–91. doi:10.1016/s0925-4927(00)00045-7
12. Petit D, Montplaisir J, Lorrain D, Gauthier S. Spectral analysis of the rapid eye movement sleep electroencephalogram in right and left temporal regions: a biological marker of Alzheimer’s disease. Ann Neurol. 1992;32(2):172–176. doi:10.1002/ana.410320208
13. Crenshaw MC, Edinger JD. Slow-wave sleep and waking cognitive performance among older adults with and without insomnia complaints. Physiol Behav. 1999;66(3):485–492. doi:10.1016/s0031-9384(98)00316-3
14. Mullins AE, Kim JW, Wong KKH, et al. Sleep EEG microstructure is associated with neurobehavioural impairment after extended wakefulness in obstructive sleep apnea. Sleep Breath. 2021;25(1):347–354. doi:10.1007/s11325-020-02066-5
15. Buysse DJ, Reynolds CF, Monk TH, Berman SR, Kupfer DJ. The Pittsburgh Sleep Quality Index: a new instrument for psychiatric practice and research. Psychiatry Res. 1989;28(2):193–213. doi:10.1016/0165-1781(89)90047-4
16. Johns MW. A new method for measuring daytime sleepiness: the Epworth sleepiness scale. Sleep. 1991;14(6):540–545. doi:10.1093/sleep/14.6.540
17. Richter P, Werner J, Heerlein A, Kraus A, Sauer H. On the validity of the Beck Depression Inventory. A review. Psychopathology. 1998;31(3):160–168. doi:10.1159/000066239
18. Beck AT, Epstein N, Brown G, Steer RA. An inventory for measuring clinical anxiety: psychometric properties. J Consult Clin Psychol. 1988;56(6):893–897. doi:10.1037/0022-006x.56.6.893
19. Jorgensen G, Downey C, Goldin J, Melehan K, Rochford P, Ruehland W. An Australasian Commentary on the AASM manual for the scoring of sleep and associated events. Sleep Biol Rhythms. 2020;18(3):163–185. doi:10.1007/s41105-020-00259-9
20. Fernandez-Mendoza J, Li Y, Vgontzas AN, et al. Insomnia is associated with cortical hyperarousal as early as adolescence. Sleep. 2016;39(5):1029–1036. doi:10.5665/sleep.5746
21. Li Y, Vgontzas AN, Fernandez-Mendoza J, Fang J, Puzino K, Bixler EO. Effect of trazodone versus cognitive-behavioural treatment on high- and slow-frequency activity during non-rapid eye movement sleep in chronic insomnia: a pilot, randomized clinical trial. J Sleep Res. 2021;30(5):e13324. doi:10.1111/jsr.13324
22. Vgontzas AN, Pejovic S, Zoumakis E, et al. Daytime napping after a night of sleep loss decreases sleepiness, improves performance, and causes beneficial changes in cortisol and interleukin-6 secretion. Am J Physiol Endocrinol Metab. 2007;292(1):E253–E261. doi:10.1152/ajpendo.00651.2005
23. Perlis ML, Smith MT, Andrews PJ, Orff H, Giles DE. Beta/Gamma EEG activity in patients with primary and secondary insomnia and good sleeper controls. Sleep. 2001;24(1):110–117. doi:10.1093/sleep/24.1.110
24. Fan J, McCandliss BD, Sommer T, Raz A, Posner MI. Testing the efficiency and Independence of attentional networks. J Cogn Neurosci. 2002;14(3):340–347. doi:10.1162/089892902317361886
25. Fan J, McCandliss BD, Fossella J, Flombaum JI, Posner MI. The activation of attentional networks. Neuroimage. 2005;26(2):471–479. doi:10.1016/j.neuroimage.2005.02.004
26. Redline S, Kirchner HL, Quan SF, Gottlieb DJ, Kapur V, Newman A. The effects of age, sex, ethnicity, and sleep-disordered breathing on sleep architecture. Arch Intern Med. 2004;164(4):406–418. doi:10.1001/archinte.164.4.406
27. Phrommintikul A, Sa-Nguanmoo P, Sripetchwandee J, Vathesatogkit P, Chattipakorn N, Chattipakorn SC. Factors associated with cognitive impairment in elderly versus nonelderly patients with metabolic syndrome: the different roles of FGF21. Sci Rep. 2018;8(1):5174. doi:10.1038/s41598-018-23550-9
28. Muhammad T, Srivastava S, Sekher TV. Assessing socioeconomic inequalities in cognitive impairment among older adults: a study based on a cross-sectional survey in India. BMC Geriatr. 2022;22(1):389. doi:10.1186/s12877-022-03076-6
29. Liscombe MP, Hoffmann RF, Trivedi MH, Parker MK, Rush AJ, Armitage R. Quantitative EEG amplitude across REM sleep periods in depression: preliminary report. J Psychiatry Neurosci. 2002;27(1):40–46.
30. Lehmann M, Schreiner T, Seifritz E, Rasch B. Emotional arousal modulates oscillatory correlates of targeted memory reactivation during NREM, but not REM sleep. Sci Rep. 2016;6:39229. doi:10.1038/srep39229
31. Diekelmann S, Born J. The memory function of sleep. Nat Rev Neurosci. 2010;11(2):114–126. doi:10.1038/nrn2762
32. Tzischinsky O, Lavie P. Melatonin possesses time-dependent hypnotic effects. Sleep. 1994;17(7):638–645. doi:10.1093/sleep/17.7.638
33. Black JE, Guilleminault C, Colrain IM, Carrillo O. Upper airway resistance syndrome. Central electroencephalographic power and changes in breathing effort. Am J Respir Crit Care Med. 2000;162(2 Pt 1):406–411. doi:10.1164/ajrccm.162.2.9901026
34. Gabryelska A, Feige B, Riemann D, et al. Can spectral power predict subjective sleep quality in healthy individuals? J Sleep Res. 2019;28(6):e12848. doi:10.1111/jsr.12848
35. Decker MJ, Tabassum H, Lin JM, Reeves WC. Electroencephalographic correlates of Chronic Fatigue Syndrome. Behav Brain Funct. 2009;5:43. doi:10.1186/1744-9081-5-43
36. Philipsen A, Feige B, Al-Shajlawi A, et al. Increased delta power and discrepancies in objective and subjective sleep measurements in borderline personality disorder. J Psychiatr Res. 2005;39(5):489–498. doi:10.1016/j.jpsychires.2005.01.002
37. Koetsier GC, Volkers AC, Tulen JH, Passchier J, van den Broek WW, Bruijn JA. CPT performance in major depressive disorder before and after treatment with imipramine or fluvoxamine. J Psychiatr Res. 2002;36(6):391–397. doi:10.1016/s0022-3956(02)00026-2
38. Kerr N, Scott J, Phillips ML. Patterns of attentional deficits and emotional bias in bipolar and major depressive disorder. Br J Clin Psychol. 2005;44(Pt 3):343–356. doi:10.1348/014466505×57755
39. Politis A, Lykouras L, Mourtzouchou P, Christodoulou GN. Attentional disturbances in patients with unipolar psychotic depression: a selective and sustained attention study. Compr Psychiatry. 2004;45(6):452–459. doi:10.1016/j.comppsych.2004.07.007
40. Gerber M, Cody R, Beck J, et al. Differences in selective attention and inhibitory control in patients with major depressive disorder and healthy controls who do not engage in sufficient physical activity. J Clin Med. 2023;12(10):3370.
41. Li Z, Wu TT, Xiong YT, et al. A pilot study on improvements in attention function in major depressive disorder after 12 weeks of escitalopram monotherapy or combined treatment with agomelatine. Front Psychiatry. 2023;14:1188175. doi:10.3389/fpsyt.2023.1188175
42. Schwert C, Stohrer M, Aschenbrenner S, Weisbrod M, Schröder A. Neurocognitive profile of outpatients with unipolar depressive disorders. J Clin Exp Neuropsychol. 2019;41(9):913–924. doi:10.1080/13803395.2019.1634180
43. Lyche P, Jonassen R, Stiles TC, Ulleberg P, Landrø NI. Attentional functions in major depressive disorders with and without comorbid anxiety. Arch Clin Neuropsychol. 2011;26(1):38–47. doi:10.1093/arclin/acq095
44. Tian Y, Du J, Spagna A, et al. Venlafaxine treatment reduces the deficit of executive control of attention in patients with major depressive disorder. Sci Rep. 2016;6:28028. doi:10.1038/srep28028
45. Han G, Klimes-Dougan B, Jepsen S, et al. Selective neurocognitive impairments in adolescents with major depressive disorder. J Adolesc. 2012;35(1):11–20. doi:10.1016/j.adolescence.2011.06.009
46. Sieb RA. A brain mechanism for attention. Med Hypotheses. 1990;33(3):145–153. doi:10.1016/0306-9877(90)90164-a
47. Miller R. Cortico-Hippocampal Interplay and the Representation of Contexts in the Brain. Vol. 17. Springer Science & Business Media; 2013.
48. Numan R. The prefrontal-hippocampal comparator: volition and episodic memory. Percept Mot Skills. 2021;128(6):2421–2447. doi:10.1177/00315125211041341
49. Tang YY, Tang R, Rothbart MK, Posner MI. Frontal theta activity and white matter plasticity following mindfulness meditation. Curr Opin Psychol. 2019;28:294–297. doi:10.1016/j.copsyc.2019.04.004
50. Taillard J, Sagaspe P, Berthomier C, et al. Non-REM sleep characteristics predict early cognitive impairment in an aging population. Front Neurol. 2019;10:197. doi:10.3389/fneur.2019.00197
51. Ujma PP, Bódizs R, Dresler M, et al. Multivariate prediction of cognitive performance from the sleep electroencephalogram. Neuroimage. 2023;279:120319. doi:10.1016/j.neuroimage.2023.120319
52. Iranzo A, Isetta V, Molinuevo JL, et al. Electroencephalographic slowing heralds mild cognitive impairment in idiopathic REM sleep behavior disorder. Sleep Med. 2010;11(6):534–539. doi:10.1016/j.sleep.2010.03.006
53. van Calker D, Biber K. The role of glial adenosine receptors in neural resilience and the neurobiology of mood disorders. Neurochem Res. 2005;30(10):1205–1217. doi:10.1007/s11064-005-8792-1
54. Zuo CL, Wang CM, Liu J, et al. Isoflurane anesthesia in aged mice and effects of A1 adenosine receptors on cognitive impairment. CNS Neurosci Ther. 2018;24(3):212–221. doi:10.1111/cns.12794
55. Ujma PP, Konrad BN, Gombos F, et al. The sleep EEG spectrum is a sexually dimorphic marker of general intelligence. Sci Rep. 2017;7(1):18070. doi:10.1038/s41598-017-18124-0
56. Zhang C, Wei L, Zeng F, et al. NREM sleep EEG characteristics correlate to the mild cognitive impairment in patients with parkinsonism. Biomed Res Int. 2021;2021:5561974. doi:10.1155/2021/5561974