Fitness
Characterization of resistant ST15-KL112 K. pneumoniae | IDR
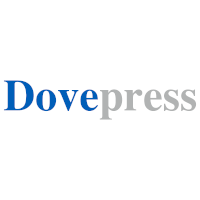
Bailong Hou,1,* Ying Zhou,2,* Wei Wang,1 Weifeng Shen,1 Qinlong Yu,1 Minjie Mao,1 Siheng Wang,1 Wenxiu Ai,3 Fangyou Yu,2,3 Pingyang Shao1
1Department of Clinical Laboratory Medicine, The First Hospital of Jiaxing, The Affiliated Hospital of Jiaxing University, Jiaxing, 314000, People’s Republic of China; 2Department of Clinical Laboratory Medicine, Shanghai Pulmonary Hospital, Tongji University School of Medicine, Shanghai, 200000, People’s Republic of China; 3Department of Clinical Laboratory, The First Affiliated Hospital of Wenzhou Medical University, Wenzhou, 325000, People’s Republic of China
*These authors contributed equally to this work
Correspondence: Pingyang Shao, Department of Clinical Laboratory Medicine, The First Hospital of Jiaxing, The Affiliated Hospital of Jiaxing University, Jiaxing, 314000, People’s Republic of China, Email [email protected] Fangyou Yu, Department of Clinical Laboratory Medicine, Shanghai Pulmonary Hospital, Tongji University School of Medicine, Shanghai, 200000, People’s Republic of China, Email [email protected]
Introduction: This study aimed to investigate the emergence and characteristics of carbapenem-resistant Klebsiella pneumoniae (CRKP) strains that demonstrate resistance to multiple antibiotics, including aminoglycosides and tigecycline, in a Chinese hospital.
Methods: A group of ten CRKP strains were collected from the nine patients in a Chinese hospital. Antimicrobial Susceptibility Testing (AST) and phenotypic inhibition assays precisely assess bacterial antibiotic resistance. Real-time quantitative PCR (RT-qPCR) was used to analyze the mRNA levels of efflux pump genes (acrA/acrB and oqxA/oqxB) and the regulatory gene (ramA). The core-genome tree and PFGE patterns were analyzed to assess the clonal and horizontal transfer expansion of the strains. Whole-genome sequencing was performed on a clinical isolate of K. pneumoniae named Kpn20 to identify key resistance genes and antimicrobial resistance islands (ARI).
Results: The CRKP strains showed high resistance to carbapenems, aminoglycosides (CLSI, 2024), and tigecycline (EUCAST, 2024). The mRNA expression levels of efflux pump genes and regulatory genes were detected by RT-qPCR. All 10 isolates had significant differences compared to the control group of ATCC13883. The core-genome tree and PFGE patterns revealed five clusters, indicating clonal and horizontal transfer expansion. Three key resistance genes (blaoxa-232, blaCTX-M-15, and rmtF) were observed in the K. pneumoniae clinical isolate Kpn20. Mobile antibiotic resistance islands were identified containing blaCTX-M-15 and rmtF, with multiple insertion sequences and transposons present. The coexistence of blaoxa-232 and rmtF in a high-risk K. pneumoniae strain was reported. Conjugation assay was utilized to investigate the transferability of blaoxa-232-encoding plasmids horizontally.
Conclusion: The study highlights the emergence of ST15-KL112 high-risk CRKP strains with multidrug resistance, including to aminoglycosides and tigecycline. The presence of mobile ARI and clonal and horizontal transfer expansion of strains indicate the threat of transmission of these strains. Future research is needed to assess the prevalence of such isolates and develop effective control measures.
Keywords: blaOXA-232, rmtF, Klebsiella pneumoniae, carbapenemase, plasmid
Introduction
Based on the data of the CHINET antimicrobial resistance surveillance program from 2005 to 2021, the resistance rates of Klebsiella pneumoniae to imipenem and meropenem increased markedly.1 The main mechanisms are carbapenemase production and efflux pumps diversification. Carbapenem-resistant Klebsiella pneumoniae (CRKP) often carry multiple drug resistance genes, the resistance rates to clinical commonly used antimicrobial agents are often more than 60%, only sensitive to tigecycline, polymyxin, and some aminoglycosides. For this class of multi-resistant or pan-resistant Enterobacteriaceae, only the more toxic polymyxin can be selected clinically, or the combination of drugs, such as the combination of tigecycline or aminoglycosides and β-lactamases.2 Tigecycline as a derivative of minocycline derivatives not only overcomes the common resistance mechanism of tetracyclines but also was found to have good antimicrobial effects on multiple resistant bacteria.3 Aminoglycoside drugs interfere with protein synthesis by binding to the highly conserved A site of 16S rRNA of the 30S ribosomal subunit of prokaryotes, leading to bacterial death.4 However, some multi-resistance, pan-resistance, and “super-bacteria” K. pneumoniae still appear, so we need to understand the resistance mechanism and transmission characteristics of these strains to provide relevant strategies for clinical treatment.
According to data collected by worldwide surveillance programs, OXA-48-like carbapenemases are the predominant type of carbapenemases found in Enterobacterales strains across different nations. There have been over twelve various forms of OXA-48-like carbapenemase identified, with OXA-232 being the third most prevalent OXA-48-like mutation worldwide at present.5 After the first identification of OXA-232-producing CRKP in five newborn patients in China in 2017,6 there have been multiple small-scale outbreaks of this strain in hospitals affecting children and elderly individuals.7 Since tigecycline was first used in clinical treatment in 2005,8 cases of drug resistance have emerged, and the most common mechanism for conferring resistance is either overexpression of non-specific active efflux pumps or mutations within ribosomal drug-binding sites.9 The mechanism of resistance to aminoglycosides is mainly for bacteria to obtain modified enzymes of aminoglycosides, such as acetyltransferase, phosphorylase and adenosine transferase, and also to obtain plasmid-mediated 16S rRNA methylase resulting in high resistance to all aminoglycoside drugs.10 RmtF is a 16S-RMTase, first found in France in 2012, and has since only occurred alone in the United States, India, and some European countries.11 RmtF was first reported in 2020 in Shanghai, China.12 Although CRKP with high levels of tigecycline or aminoglycosides resistance appears. However, CRKP with concurrent resistance to both tigecycline and aminoglycosides was rarely reported.
In our study, we found ten OXA-232-producing K. pneumoniae strains appearance in a tertiary hospital, which occurred from July 2020 to January 2021. These strains were simultaneously resistant to carbapenems, tigecycline, as well as aminoglycosides. We characterized the resistance characteristics and analyzed the genetic evolutionary relationships and related resistance transmission mechanisms. The aim of this study is to describe the resistance mechanisms and transmission patterns of these high-risk bacteria.
Materials and Methods
Bacterial Isolates and Identification
A group of ten CRKP strains were collected from the nine patients in the Affiliated Hospital of Jiaxing University (Zhejiang, China) during July 2020 to January 2021. The strains were K. pneumoniae 7.1/ 7.2/ 11/ 12/ 13/ 15/ 16/ 17/ 20/ 30, and Kpn 7.1 / 7.2 strains morphology were different but come from the same patient The bacteria obtained from the culture were preserved in glycerol broth at a temperature of −80°C. Specimens were placed on Colombian blood Agar plates and then analyzed using matrix-assisted laser desorption/ionization time of flight mass spectrometry (MALDI-TOF MS) following the guidelines provided by the manufacturer for identification. Clinical data were acquired from the Electronic Medical Records of the patients and the study were approved by the Ethical Committee of the Affiliated Hospital of the Jiaxing University. Escherichia coli American type culture collection (ATCC) 25,922 and Pseudomonas aeruginosa ATCC27853 were used as control strains for the identification and antimicrobial susceptibility test (AST). E. coli ATCC13883 was used as control strain for the quantitative real-time PCR (RT-qPCR) test.
Antimicrobial Susceptibility Testing
A total of 19 antimicrobial agents were tested, including imipenem (IPM), meropenem (MEM), ertapenem (ETP), amoxicillin/clavulanic acid (AMC), piperacillin-tazobactam (TZP), cefuroxime (CXM), cefuroxime axetil, cefoperazone/sulbactam (SCF), ceftriaxone (CRO), ceftazidime (CAZ), cefepime (FEP), cefoxitin (FOX), gentamicin (GEN), amikacin (AMK), Tobramycin (TOB), ciprofloxacin (CIP), levofloxacin (LEV), sulfamethoxazole (SXT) and tigecycline (TGC). Minimum inhibitory concentrations (MICs) for the tested bacteria were determined by using either the agar dilution method or broth microdilution method to assess the minimum inhibitory concentrations of antimicrobial agents. We interpreted the susceptibility breakpoints following the guidelines of the Clinical and Laboratory Standards Institute (CLSI 2024), except for tigecycline, for which we utilized the European Committee on Antimicrobial Susceptibility Testing (EUCAST, 2024) breakpoints.
Tigecycline Resistance and Phenotypic Inhibition Test
The MIC of tigecycline was determined by broth microdilution method. MIC results were interpreted according to the (EUCAST, 2024) clinical breakpoints (for tigecycline, ≤0.5 mg/L is susceptible, and >0.5 mg/L is resistant). E. coli ATCC 25922 was used for quality control in the susceptibility assays. Isolates that showed resistance to tigecycline also tested by adding efflux pump inhibitor Carbonyl cyanide m-chlo rophenylhy’drazone (CCCP) to the medium.
Real-Time Quantitative PCR (RT-qPCR)
RT-qPCR was used to analyze the mRNA levels of efflux pump genes (acrA/acrB and oqxA/oqxB) and their regulators (ramA). Overnight bacterial cultures were diluted 1/100 in Luria-Bertani (LB) broth (Sigma-Aldrich, USA) and grown to log phase at 37°C with vigorous shaking (220 rpm). Total RNA was extracted from the cells, and cDNA was synthesized using an RNA PCR kit (Takara Bio, Otsu, Japan). The yield and quality of RNA and cDNA were determined using a Nanodrop 2000C (Thermo, USA). Q-PCR was performed in a 20 µL reaction volume using Fast Start DNA Master SYBR Green II Mixture (Takara, Tokyo, Japan). A housekeeping gene (rpoB) was the internal reference in the RT-qPCR. The expression levels of each target gene were compared to the expression level of a tigecycline-susceptible E. coli ATCC13883 isolate (expression = 1), which was used as the reference sample. Analysis was conducted utilizing the 2−ΔΔCT technique.The experiments were conducted three times.
Statistical Analysis
The comparison of statistical significance between the two strains was conducted using a two-tailed Student’s t-test with Bonferroni correction in the GraphPad Prism 6 program. A p-value less than 0.05 was deemed to be statistically significant.
Pulsed Field Gel Electrophoresis (PFGE) and S1 Pulsed-Field Gel Electrophoresis (S1-PFGE)
Genetic relatedness was assessed through molecular typing with PFGE. Samples with genomic DNA digested by XbaI were loaded into wells and subjected to electrophoresis for 17.5 hours at 6 volts per centimeter at 14 degrees Celsius using the Bio-Rad® CHEF-Mapper XA apparatus (Bio-Rad, California, USA). The pulse durations at the beginning and end were 6 seconds and 36 seconds, respectively. Salmonella enterica strain Braenderup H9812 was used as the indicator.
Plasmid profiles were obtained from 10 CRKP isolates producing OXA-232 using S1-PFGE. In short, the samples were enclosed in a Seakem Gold gel solution at a concentration of 10 g/L, treated with endonuclease S1 nuclease from Takara in Dalian, China, and then analyzed using pulsed-field gel electrophoresis under specific conditions (14°C, 6 V/cm, 120◦ electric field angle, 4.0–40 s conversion time, and 19 h electrophoresis). Genomic DNA from the Salmonella enterica serovar Braenderup H9812 strain was digested with XbaI and utilized as a reference strain and molecular weight marker.
Whole Genome Sequencing (WGS) and Bioinformatics Analysis
Bacterial genomic DNA was isolated using the UltraClean Microbial Kit (Qiagen, NW, Germany) and sequenced from a sheared DNA library with average size of 15 kb (ranged from 10 to 20 kb) on a PacBio RSII sequencer (Pacific Biosciences, CA, United States), as well as a paired-end library with an average insert size of 350 bp (ranged from 150 to 600 kb) on a HiSeq sequencer (Illumina, CA, United States). Sequencing libraries were constructed using the NEBNext RUltraTM II DNA Library Prep Kit for Illumina R (second-generation sequencing) and the SMRTbell RExpress Template Prep Kit 2.0 (third generation sequencing) and then loaded onto NovaSeq S4 flowcell and SMRT Cell 8 M DNA sequencing chip, respectively. Paired-end short Illumina reads were employed for correcting the long PacBio reads with proovread, followed by de novo assembly of the corrected PacBio reads using SMARTdenovo (https://github.com/ruanjue/smartdenovo). ResFinder 3.2 from the Center for Genomic Epidemiology (https//cge.cbs.dtu.dk/services/ResFinder/) was used to detect antimicrobial resistance genes. PlasmidFinder 2.1, pMLST 2.0, and MLST 2.0 software were used to determine the plasmid incompatibility groups, pMLST, and multilocus sequence typing (MLST). We checked if the plasmid was a conjugative plasmid by analyzing the conjugation module using the oriT Finder website (https//bioinfo-mml.sjtu.edu.cn/oriTfifinder/). The IS elements can be identified directly from the established website (https//www-is.biotoul.fr/). We utilized blast (https//blast.ncbi.nlm.nih.gov/Blast.cgi) to identify plasmids with similarities through comparison of their coverages and identities. The circular representation of pKpn20 was generated with CGview (http://stothard.afns.ualberta.ca/cgview_server/). The plasmid linear graph was analyzed by Easyfig software (http://mjsull.github.io/Easyfifig/). Prophages, repeated regions, and recombination regions were screened for single nucleotide polymorphisms (SNP), and the core SNP variations were identified with SNP-dists (https//github.com/tseemann/snp-dists).
Conjugation Experiment
Conjugation assay was utilized to investigate the transferability of blaOXA-232-encoding plasmids horizontally. The Kpn20 served as the donor strain, while the recipient strain was E. coli J53 (resistant to sodium azide). Both the contributors and beneficiaries were grown to the logarithmic stage (OD600 = 0.4–0.6), combined in equal parts, spun at 12,000 rpm for 1 minute, reconstituted in 20 µL of LB broth, and left to grow overnight at 37°C. Next, the spots were moved to 15 mL tubes for centrifugation and rinsed with 3 mL of LB broth. Subsequently, the serial dilutions were plated onto MH agar plates containing Ampicillin (100 µg/mL) and sodium azide (100 µg/mL). Donor and recipient cells were each utilized as controls to confirm the screening plate antibiotics’ efficacy. The K. pneumoniae 1632 was the Positive control in the test.13 PCR was used to confirm the presence of blaOXA-232 in all transconjugants. The transconjugants underwent susceptibility testing. Conjugation frequency was determined by counting the amount of transconjugants that were produced for each recipient.
Results
The Clinical Characterization of Klebsiella Pneumoniae Co-Harboring Blaoxa-232 and rmtF
Between July 1th 2020 to January 30th 2021, ten OXA-232-producing K. pneumoniae strains were detected from a tertiary hospital in China. The ten strains were isolated from nine patients. These strains were recovered from sputum (n=8) and blood (n=2).
These patients were admitted into the ICU ward or the neurosurgery ward, and most patients share the same ward at the same time (Table 1 and Figure 1). It is worth that the two wards are located on the third and fifth floors of the same building (the fourth floor no patients), and the frequent movement of humans (medical personnel, patients, and visitors) and portable medical equipment occurs between the two floors, providing many opportunities for bacterial pathogens to spread between wards in hospitals. The mean age of these patients was 68 years, the majority of whom were men (67%). It is noteworthy that most patients have severe underlying disease, including hypertension, heart disease and renal inadequacy et al. The majority of patients were treated with β-lactam antibiotics, such as piperacillin/tazobactam, meropenem, tigecycline, and cefoperazone/sulbactam. Most patients underwent invasive surgery and instrumentation, including peripherally inserted central catheterization, evacuation of meninges hematoma and tracheotomy et al (Table 1). Furthermore, some patients had a poor prognosis, and six patients died during their hospital stay. Other clinical characteristics of these nine patients were summarized and listed in Table 1.
Table 1 Clinical Features of Patients Infected with OXA-232-Producing K. Pneumoniae Isolates |
Antibiotic Resistance Phenotypes
High resistance to cephalosporins, fluoroquinolone, and aminoglycoside was observed in the antibiotic susceptibility test for K. pneumoniae, with the exception of a single strain (Kpn30) that showed susceptibility to aminoglycoside. Various strains exhibited varying degrees of resistance to different types of carbapenems, including imipenem, meropenem, and ertapenem. Every strain showed resistance to ertapenem with minimum inhibitory concentrations (MICs) equal to or greater than 8 μg/mL, however, they exhibited varying degrees of resistance to meropenem (MICs between 2 and 4 μg/mL) and imipenem (MICs between 1, 2 and 4 μg/mL) as shown in Table 2.
![]() |
Table 2 In vitro Activities of Antimicrobial Agents Against OXA-232-Producing K. Pneumoniae Isolates (MICs μg/Ml) |
Overexpression of Efflux Pump Mediated Tigecycline-Resistant
Of the ten OXA-232-producing K. pneumoniae isolates were resistant to tigecycline (MICs ranged from 2 to 8 μg/mL). The MIC distribution is presented in Table 2. The tigecycline resistance rate for these strains was 100% (MIC>0.5 mg/L, EUCAST, 2024). Exposure of tigecycline-resistant isolates to the efflux pump inhibitor CCCP resulted in an obvious decrease (1 to 2-fold) in the MICs of tigecycline for the strains (Kpn 13 and Kpn 30) but do not restored susceptibility.
mRNA expression levels of efflux pump genes (acrA/acrB and oqxA/oqxB) and regulators (ramA) were examined by RT-qPCR. Primers used RT-qPCR studies and PCR amplification. Figure 2 depicts the results of RT-QPCR experiments on efflux pump genes in various isolates and the ATCC13883 control group. The Figure 2 comprises three parts: A, B, and C, and each panel includes statistical significance indicators. In Figure 2A, the expression levels of efflux pump genes acrA and acrB are presented. The 10 isolates are compared to control group ATCC13883, with varying expression levels indicated numerically. The statistical significance of the differences in gene expression levels between the control and each isolates is denoted (*pFigure 2B focuses on the efflux pump genes oqxA and oqxB. Similar to Figure 2A, statistical significance indicators are provided to indicate which isolates exhibit significant changes in gene expression. In Figure 2C highlights the efflux pump regulatory gene ramA. Statistical significance is also assessed for ramA expression. The findings indicated a significant correlation between mRNA levels of efflux pump genes and tigecycline MICs (p
Molecular Epidemiology and Characteristics Analysis of blaOXA-232-Producing K. Pneumoniae
Whole-genome sequencing of the genomic DNA from ten K. pneumoniae strains revealed that they were part of the widespread clone ST15 and had capsular type KL112, a prevalent global variant linked to various β-lactamases such as OXA-232, CTX-M-15, SHV-106, TEM-1B, and SHV-28 (Table 3).
![]() |
Table 3 Gene Characteristics of ten OXA-232-Producing CRKP Isolates |
The core-genome tree and PFGE patterns showed that strains formed five clusters (Figure 3). The S1-PFGE experiment result showed that every K. pneumoniae isolates have multiple plasmids. Plasmids in different strains have similar properties. The Kpn20 was the first CRKP clinical strain producing OXA-232, which can be found that OXA-232-producing CRKP from July 2020 until a small outbreak began in October. All blaOXA-232-positive K. pneumoniae isolates were low-level resistance to carbapenems, and isolates which were positive for blaCTX-M-15 and rmtF showed high-level drug resistance to aminoglycosides except Kpn30. In particular, strains Kpn 12 and Kpn 13 showed extremely close relationships in both PFGE (Figure 3A), which are also evident in the phylogenetic tree (Figure 3C). Strains Kpn 7.1, Kpn 7.2 and Kpn 11 also show extremely close relationships in PFGE (Figure 3A), which is also evident in the phylogenetic tree (Figure 3C). The kpn 7.1 and kpn 7.2 strains morphology were different but come from the same patient (Figure 3D). From the structure of the phylogenetic tree, strains Kpn 12 and Kpn 13 are closely linked, as are Kpn 7.1, Kpn 7.2 and Kpn 11, each forming a separate subgroup. This close relatedness may imply a high degree of similarity in genetic composition and genetic characteristics. In terms of the clinical patient factor analysis, we noted some similarity between the isolation sources of strain Kpn 12 and Kpn 13. Specifically, both strains were from the same ward of the same hospital in the same region and were isolated at close times, both isolated from clinical patients with invasive surgical procedures. Similarly, strains Kpn 7.1, Kpn 7.2 and Kpn 11 isolate origins are quite similar. This similarity of clinical information further suggests some possible route of transmission or cross-infection between strains Kpn 12 and Kpn 13 and between strains Kpn 7.1, Kpn 7.2 and Kpn 11.
Plasmid Structure Analysis
Every CRKP that produced OXA-232 also contained the ESBL gene blaCTX-M-15. The failed transfer of the plasmid containing blaOXA-232 to the J53 strains in conjugation trials indicated that the plasmid carrying blaOXA-232 did not exhibit strong conjugation abilities.
Nanopore long-read sequence was used for the Kpn20, which was the first CRKP clinical strain producing OXA-232. Kpn20 strain contained seven plasmids (Table 4). Similar plasmids were not found in the NCBI database, and it is possible that the Kpn20 strain was integrated into new large plasmids later in their evolution.
![]() |
Table 4 General Features, Antimicrobial Resistance Genes of Plasmids in Kpn20 |
WGS results showed that there were two important plasmids, blaOXA-232-carrying plasmid (pKPN20-5) and rmtF-carrying plasmid (pKPN20-1), respectively. Plasmid pKPN20-1 with rmtF and blaCTX-M-15 (Figure 4A) was a 212,639-bp circular molecule and an average GC content of 51.62% (Table 4). Compared with the p47733_CTX_M_15 (GenBank no. CP050364.1) plasmid.14 The two plasmids have a very low similarity, only having roughly about 90% similarity in the environment around the blaCTX-M-15 gene, and less than 10% similarity elsewhere. Plasmid pKPN20-5 with blaOXA-232 (Figure 4B) had a length of 6141 bp and an average GC content of 52.17% (Table 4). Plasmid pKPN20-5 was highly similar to the pKNICU5 (GenBank no. KY454616.1) plasmid, which previously isolated from K. pneumoniae in Chinese children. Moreover, we analyzed the peripheral gene structure characterized of the blaCTX-M-15-harboring plasmid (Figure 4C).And the rmtF-harboring plasmid (Figure 4D) in this study. The gene sequence maps provides a detailed visualization of a multi-gene family, encompassing multiple insertion sequence (IS) genes distinguished by varying colors and labels. These colors represent the polymorphism or differences among the genes. The map also includes genes related to antibiotic resistance, transposases, type IV secretion systems, integrases, and virulence factors, all of which play crucial roles in bacterial drug resistance, gene rearrangement, DNA transfer, and infection capabilities. Additionally, the map highlights hypothetical protein-coding genes with undefined functions, suggesting the need for further research to uncover their potential roles.
Discussion
The emergence of Klebsiella pneumoniae that produce carbapenemase poses a significant obstacle to antimicrobial treatment, but the mortality rate can be reduced effectively through combination therapy with aminoglycosides and tigecycline. However, we monitored an outbreak of high-risk Klebsiella pneumoniae co-carrying blaOXA-232 and rmtF. Notably, these strains exhibited multidrug resistance, with resistance to carbapenems, aminoglycosides and tigecycline. Our study characterized the resistance characteristics and analyzed the genetic evolutionary relationships and related resistance transmission mechanisms of this strains to provide a basis for controlling the further spread of such multidrug-resistant bacteria. Future studies are necessary to evaluate the prevalence of such isolates.
In our study the first OXA-232-carrying strain was isolated in July 2020 and the patient was admitted to the ICU (3rd floor) with chest wall swelling and discomfort, and the CRKP isolate was detected in the patient’s sputum after 55 days of hospitalization. The second OXA-232-carrying strain was isolated in October 2020, and the patient was admitted to the neurosurgical ward (5th floor) after progression of disease and transferred to the ICU (3rd floor). Subsequently, from November 2020 to January 2021, seven more CRKP strains were detected in the same ICU and neurosurgery ward (Figure 1).
During the COVID-19 pandemic in 2020–2021, the transmission of CRKP strains co-harboring blaoxa-232 and rmtF significantly intensified, primarily attributed to a combination of factors including the increased bacterial resistance resulting from prolonged antibiotic usage in hospitals, elevated risk of cross-infection within healthcare facilities, inappropriate antibiotic use, and decreased immune function among patients. The combination of these elements presented a major danger to public health by aiding in the transmission of CRKP and other pathogens commonly found in healthcare settings. Due to the current COVID-19 pandemic, hospitals are implementing stricter infection control measures, such as chlorhexidine skin cleansing for patients, increased hand hygiene among healthcare workers, ensuring availability of hand hygiene supplies, limiting hospital visitors, cleaning patients’ surroundings, and improving disinfection of medical equipment. These measures also helped to control the prevalent of blaOXA-232-carrying strains.
Recent publication reported during 2013–2021, increased prevalence of OXA-232-producing Enterobacterales was ST14 K. pneumoniae isolates in France.15 But from 2018 to 2021, ST15 K. pneumoniae isolates with production of OXA-232 is the most prevalent OXA-48-like derivative in Zhejiang, China.16 In this study, we described the prevalent of ten K. pneumoniae strains recovered from the patients in a tertiary hospital in China. This prevalent was caused by ST15-KL112 K. pneumoniae which carried blaOXA-232 and rmtF. These patients were mainly hospitalized in the same building, and most patients share the same ward at the same time. Since the first report of an OXA-232-producing K. pneumoniae strain in China in 2016, such isolates have become epidemic in China, and usually associated with a clonal dissemination of ST15 K. pneumoniae. An important feature of carbapenem-resistant organisms (CRO) with blaOXA-232 on the pOXA-232-like plasmids is its different geographical distribution.17 For example, ST14 was mainly in Western Canada18 and the Burn Intensive Care Unit in South Korea.19 ST16 was mainly reported from three Italian institutions.20 ST17 was mainly in US hospitals21 and ST231 was mainly in Switzerland.22 As the second prevalent strain of OXA-232-producing K. pneumoniae in China, ST15 strain is of great harm, so we need to control its outbreak prevalence in local hospitals.
The spread of mobile genetic elements like plasmids, phages, integrations, conjugative elements, and insertion elements plays a crucial role in the prevalence of K. pneumoniae. In this study, we found that each strain carried multiple plasmids, and the number and size were similar with the first strain Kpn20, so Kpn20 was described in detail to better understand the characteristics of drug-resistant plasmids.23
Potron A. and team discovered that the gene blaOXA-232, which confers resistance to carbapenems, was situated on a compact plasmid named pOXA-232 with a size of 6141 base pairs, and it contained a backbone similar to ColE.24 Plasmid pOXA-232 was not capable of conjugation.25,26 The plasmid found in our experimental strains closely resembled those observed in previous research. The attempt to transfer blaOXA-232 into E. coli through conjugation was unsuccessful, suggesting that the plasmid was not highly conjugative. Zhang et al discovered that the transfer of ColKP3-type plasmid to E. coli emphasized the significance of comprehending the transmission process in order to prevent or stop the spread of blaOXA-232 to different organisms.16
Most of the strains in this study also carried blaCTX-M-15 and rmtF that mediated high-level resistance to aminoglycosides. Most studies have found that blaCTX-M-15 and rmtF were located on two different plasmids, but in this study these two genes co-located in one plasmid, named pKPN20-1, and no similar plasmid was found in NCBI database. We speculate that pKPN20-1 may have been integrated into a large plasmid during evolution with the mobilization of IS elements and transposons surrounding with the blaCTX-M-15 and rmtF.
Our findings offer fresh perspective on the correlation between genetic analysis and medical therapy. Increased monitoring of blaOXA-232 is urgently required in China, as they suggest.
But this study has several limitations. First, only one hospital was selected. Therefore, these findings should not be extrapolated to hospitals with a wide variety in CRO admission rates. Second, the collection time of patients and isolates was not long enough. The epidemiology of CRO in other parts of China may be substantially different.
Conclusion
This study describes a nosocomial prevalent of ST15-KL112 K. pneumoniae with co-carrying blaOXA-232 and rmtF in a tertiary hospital from July 2020 to January 2021. This epidemic is not mediated by clonal spread, it results from the horizontal spread of two resistant plasmids by non-conjugation means. Hence, immediate implementation of infection prevention measures is crucial to avoid a future outbreak in the area. Multiple mobile elements are crucial in acquiring antibiotic resistance and in plasmid evolution. Further research is needed to assess how common these plasmids are in clinical samples from China and other nations.
Data Sharing Statement
The complete nucleotide sequences of the pKPN20-1, pKPN20-2, pKPN20-3, pKPN20-4, pKPN20-5, pKPN20-6 and pKPN20-7 were deposited as GenBank accession numbers CP092337, CP092338, CP092339, CP092340, CP092341, CP092342 and CP092343, respectively.
The datasets presented in this study can be found in online repositories. The names of the repository/repositories and accession number(s) can be found in the article material.
Ethics Approval
Approval for the study was granted by the ethics committee at Jiaxing’s First Hospital (No.2023-LY-205). The microbiology hospital laboratory provided anonymous patient information, including the isolated strains. The research adhered fully to the principles outlined in the Declaration of Helsinki.
Acknowledgments
We thank anonymous reviewers whose comments and suggestions helped improve this manuscript.
Funding
Funding for this research was provided by the Science and Technology Plan Project of Jiaxing in Zhejiang Province, China (Grant 2022AD30056) and the Clinical Laboratory Medical Diagnostics Fund of the First Hospital of Jiaxing in Zhejiang Province, China (Grant 2023-ZC-002).
Disclosure
The authors affirm that the study was carried out without any business or monetary affiliations that could be interpreted as a possible clash of interests.
References
1. Ding L, Guo Y, Hu F. Antimicrobial resistance surveillance: china’s nearly 40-year effort. Int J Antimicrob Agents. 2023;62:106869. doi:10.1016/j.ijantimicag.2023.106869
2. Gao L, Lv Y, Li Y. Analysis of the drug resistance of carbapenem-resistant Klebsiella pneumoniae in the China antimicrobial resistance surveillance trial program, 2007-2018. Microb Drug Resist. 2020;26:944–950. doi:10.1089/mdr.2019.0299
3. Mastrolia M, Galli L, De Martino M, Chiappini E. Use of tigecycline in pediatric clinical practice. Exp Rev Anti-Infective Ther. 2017;15:605–612. doi:10.1080/14787210.2017.1318064
4. Lee N, Kang W, Kwon Y, et al. Chemo-enzymatic synthesis of pseudo-trisaccharide aminoglycoside antibiotics with enhanced nonsense read-through inducer activity. ChemMedChem. 2023;18:e202200497. doi:10.1002/cmdc.202200497
5. Pitout J, Peirano G, Kock M, Strydom K, Matsumura Y. The global ascendency of OXA-48-type carbapenemases. Clin Microbiol Rev. 2019;33:102–119. doi:10.1128/CMR.00102-19
6. Yin D, Dong D, Li K, et al. Clonal dissemination of OXA-232 carbapenemase-producing Klebsiella pneumoniae in neonates. Antimicrob Agents Chemother. 2017;61:385.
7. Shu L, Dong N, Lu J, et al. Emergence of OXA-232 carbapenemase-producing Klebsiella pneumoniae That Carries a pLVPK-like virulence plasmid among elderly patients in China. Antimicrob Agents Chemother. 2019;63:2246. doi:10.1128/AAC.02246-18
8. Babinchak T, Ellis-Grosse E, Dartois N, Rose G, Loh E. The efficacy and safety of tigecycline for the treatment of complicated intra-abdominal infections: analysis of pooled clinical trial data. Clin Infect Dis. 2005;41 Suppl 5:S354–S367.
9. Mao Y, Shi Q, Zhang P, Jiang Y, Yu Y. Effect of ramR loss-of-function insertion on tigecycline resistance in clinical isolates of carbapenem-resistant Klebsiella pneumoniae. J Global Antimicrob Resist. 2020;21:410–413. doi:10.1016/j.jgar.2020.01.012
10. Ma X, Fu S, Wang Y, et al. Proteomics study of the synergistic killing of tigecycline in combination with aminoglycosides against carbapenem-resistant Klebsiella pneumoniae. Front Cell Infec Microbiol. 2022;12:920761. doi:10.3389/fcimb.2022.920761
11. Galimand M, Courvalin P, Lambert T. RmtF, a new member of the aminoglycoside resistance 16S rRNA N7 G1405 methyltransferase family. Antimicrob Agents Chemother. 2012;56:3960–3962. doi:10.1128/AAC.00660-12
12. Shi Q, Han R, Guo Y, et al. Emergence of ST15 Klebsiella pneumoniae clinical isolates producing plasmids-mediated RmtF and OXA-232 in China. Infect Drug Resist. 2020;13:3125–3129. doi:10.2147/IDR.S257298
13. Zhou Y, Ai W, Guo Y, et al. Co-Occurrence of Rare ArmA-, RmtB-, and KPC-2-encoding multidrug-resistant plasmids and hypervirulence iuc operon in ST11-KL47. Klebs Pneum Microbiol Spect. 2022;10:e0237121. doi:10.1128/spectrum.02371-21
14. Piza-Buitrago A, Rincón V, Donato J, et al. Genome-based characterization of two Colombian clinical Providencia rettgeri isolates co-harboring NDM-1, VIM-2, and other β-lactamases. BMC Microbiol. 2020;20:345. doi:10.1186/s12866-020-02030-z
15. Emeraud C, Birer A, Girlich D, et al. Polyclonal Dissemination of OXA-232 Carbapenemase-Producing Klebsiella pneumoniae, France, 2013-2021. Emerg Infect Dis. 2022;28:2304–2307. doi:10.3201/eid2811.221040
16. Zhang Y, Yang X, Liu C, et al. Increased clonal dissemination of OXA-232-producing ST15 Klebsiella pneumoniae in Zhejiang, China from 2018 to 2021. Infect Diseases Poverty. 2023;12:25. doi:10.1186/s40249-023-01051-w
17. Wang M, Earley M, Chen L, et al. Clinical outcomes and bacterial characteristics of carbapenem-resistant Klebsiella pneumoniae complex among patients from different global regions (CRACKLE-2): a prospective, multicentre, cohort study. Lancet Infect Dis. 2022;22:401–412. doi:10.1016/S1473-3099(21)00399-6
18. Mataseje L, Boyd D, Fuller J, et al. Characterization of OXA-48-like carbapenemase producers in Canada, 2011-14. J Antimicrob Chemother. 2018;73:626–633. doi:10.1093/jac/dkx462
19. Jeong S, Lee K, Lee J, et al. Clonal and horizontal spread of the blaOXA-232 gene among Enterobacteriaceae in a Korean hospital. Diagn Microbiol Infect Dis. 2015;82:70–72. doi:10.1016/j.diagmicrobio.2015.02.001
20. Espinal P, Nucleo E, Caltagirone M, et al. Genomics of Klebsiella pneumoniae ST16 producing NDM-1, CTX-M-15, and OXA-232. Clin Microbiol Infec. 2019;25:385.e1–385.e5. doi:10.1016/j.cmi.2018.11.004
21. Yang S, Hemarajata P, Hindler J, et al. Evolution and transmission of carbapenem-resistant Klebsiella pneumoniae Expressing the blaOXA-232 gene during an institutional outbreak associated with endoscopic retrograde cholangiopancreatography. Clinl Infect Dis. 2017;64:894–901. doi:10.1093/cid/ciw876
22. Mancini S, Poirel L, Tritten M, Lienhard R, Bassi C, Nordmann P. Emergence of an MDR Klebsiella pneumoniae ST231 producing OXA-232 and RmtF in Switzerland. J Antimicrob Chemother. 2018;73:821–823. doi:10.1093/jac/dkx428
23. Zhu J, Li Q, Li X, et al. Successful control of the first carbapenem-resistant Klebsiella pneumoniae outbreak in a Chinese hospital 2017-2019. Antimic Resist Infec Cont. 2020;9:91. doi:10.1186/s13756-020-00757-y
24. Potron A, Rondinaud E, Poirel L, et al. Genetic and biochemical characterisation of OXA-232, a carbapenem-hydrolysing class D β-lactamase from Enterobacteriaceae. Int J Antimicrob Agents. 2013;41:325–329. doi:10.1016/j.ijantimicag.2012.11.007
25. Weng X, Shi Q, Wang S, Shi Y, Sun D, Yu Y. The Characterization of OXA-232 Carbapenemase-Producing ST437 Klebsiella pneumoniae in China. Can J Infect Dis Med Microbiol. 2020;2020:5626503. doi:10.1155/2020/5626503
26. Abdul Momin M, Liakopoulos A, Phee L, Wareham D. Emergence and nosocomial spread of carbapenem-resistant OXA-232-producing Klebsiella pneumoniae in Brunei Darussalam. J Global Antimicrob Resist. 2017;9:96–99. doi:10.1016/j.jgar.2017.02.008