Fitness
Circulating Inflammatory Proteins & Obstructive Sleep Apnea | NSS
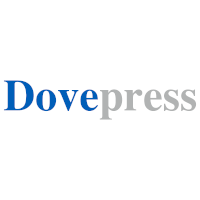
Introduction
The fundamental attributes of obstructive sleep apnea (OSA) encompass the recurrent obstruction of the upper airway during sleep, resulting in periodic hypoxemia, disturbances in sleep continuity, and increased respiratory exertion. Consequently, this sequence induces activation of the sympathetic nervous system, oxidative stress, and the emergence of systemic inflammatory responses.1 Epidemiological research indicates that approximately 936 million adults aged 30 to 69 globally are afflicted with OSA.2 With a prevalence of approximately 22% in males and 17% in females, OSA stands as a prevalent sleep breathing disorder in clinical contexts.3 A comprehensive comprehension of the pathophysiological mechanisms governing OSA is imperative for effective clinical intervention.
The systemic inflammatory cascade is thought to assume a crucial role in both the commencement and development of OSA.4 Prior clinical investigations have explored the plasma concentrations of inflammatory markers in both OSA patients and controls, uncovering elevated levels of plasma high-sensitivity C-reactive protein (hsCRP), interleukin-6 (IL-6), tumor necrosis factor-alpha (TNF-α), and pentraxin-3 (PTX-3) in individuals with OSA.5 Nonetheless, a separate study found no statistically significant differences in serum levels of IL-6 (p = 0.782) and TNF-α (p = 0.722) between OSA patients and the controls. Furthermore, continuous positive airway pressure (CPAP) treatment demonstrated no significant impact on IL-6 and TNF-α levels.6 The Multicenter Obstructive Sleep Apnea Interventional Cardiovascular (MOSAIC) trial conducted a comparative analysis of inflammatory markers between a 6-month CPAP treatment group and an untreated OSA patient group. The results revealed no substantial differences in plasma IL-6, interleukin-10 (IL-10), C-reactive protein (CRP), or TNF-α levels, irrespective of CPAP adherence.7 A separate study exploring the relationship between specific hematological and polysomnographic parameters in OSA patients revealed independent associations between albumin, neutrophil, and monocyte counts, as well as the systemic inflammation response index (SIRI), with reduced oxygen saturation levels in OSA patients.8
Mendelian randomization (MR) is a modern research methodology that utilizes genetic variations strongly linked to exposure factors as instrumental variables (IVs). It aims to infer causal relationships between exposure and outcome. Rooted in Mendel’s law of random allele distribution during meiosis, this approach significantly reduces the influence of confounding factors and reverse causality, allowing for more meaningful causal inferences compared to traditional observational studies.9 Moreover, large-scale clinical studies face challenges related to ethics, manpower, and financial resources. MR, to some extent, addresses these challenges. Two-sample MR analysis, as opposed to single-sample MR analysis, allows researchers to evaluate the relationship between exposure and outcome in two distinct population samples, thereby bolstering the credibility and effectiveness of research outcomes.10 Previous studies have employed MR analysis to identify novel genome-wide significant loci associated with OSA and objective sleep traits, illuminating the genetic architecture of OSA.11 Furthermore, another investigation unveiled a significant genetic correlation between OSA and BMI.12 MR methods have also been utilized to explore the association between OSA and inflammatory markers. Nevertheless, these investigations were not exhaustive. Minhan Yi et al conducted a causal analysis examining the correlation between nine interleukins and OSA, revealing no significant causal relationship, indicating the need for further investigation.13 Another study by the same team investigated the association and causal relationship between CRP and TNF-α levels and OSA. The researchers found that elevated levels of CRP and TNF-α were associated with OSA severity and exhibited a positive response to CPAP therapy. Additionally, OSA demonstrated a potentially causal effect on elevated CRP levels.14
In summary, existing clinical studies investigating inflammatory marker levels in OSA patients lack consensus, yielding conflicting results. These discrepancies may arise from subtle variations in study populations and methodologies. Furthermore, constraints in sample size and the influence of confounding factors have added complexity to research in this domain. Nonetheless, there has been limited research on the application of MR methods to explore the association between OSA and inflammation. Inflammation may involve alterations in multiple indicators, a facet not fully addressed in previous studies. Therefore, based on previous research, we undertook a more thorough investigation. Considering potential inflammatory biomarkers that may contribute to the development and progression of OSA, we utilized summary data from genome-wide association studies (GWAS) and employed MR methods for bidirectional causal analysis. This approach aimed to elucidate the intricate relationship between OSA and inflammatory biomarkers within the context of inflammatory cascades.
Materials and Methods
Study Design
MR rests on three foundational assumptions: First, relevance, asserting a robust correlation between the chosen genetic variations as IVs and the exposure factors; Second, independence, positing that genetic variations are unrelated to confounding factors; Third, exclusion restriction, affirming that genetic variations can solely influence outcomes through exposure.15
In order to identify potential positive inflammatory markers associated with OSA as comprehensively as possible for future exploratory research and further validation. This study conducted a comprehensive screening of GWAS data on inflammation-related markers, considering factors such as timeliness, availability, and study population. Eventually, data from GWAS of 91 newly published circulating inflammatory proteins (CIPs) was selected.
This investigation utilized publicly available summary-level data from 91 CIPs and OSA GWAS, with the populations being of European descent. To scrutinize the causal relationships between OSA and CIPs, we implemented a bidirectional MR, as depicted in Figure 1. In the forward MR, with OSA as the outcome, we chose genetic variations associated with each circulating inflammatory protein to deduce the causal associations between 91 CIPs and OSA. Subsequently, in the reverse MR, with CIPs as the outcome, we utilized genetic variations linked to OSA to infer the causal connections between OSA and 91 CIPs.
Data Source
In preceding investigations, a Genome-wide Proteome Quantitative Trait Loci (pQTL) analysis was executed on 91 CIPs across 11 cohorts encompassing a total of 14,824 participants.16 The summary-level GWAS of all 91 CIPs are available for download from the EBI GWAS Catalog, with IDs ranging from GCST90274758 to GCST90274848.
Summary-level data pertaining to OSA was extracted from a comprehensive European population-wide GWAS sourced from the FinnGen biobank project (www.finngen.fi/en).17 We opted for the publicly available summary-level OSA data, specifically the R9 version, encompassing 38,998 cases of OSA patients and 336,659 cases of the control group. The diagnosis of OSA was grounded in the International Classification of Diseases codes (ICD-10: G47.3; ICD-9: 3472A) and confirmed through subjective symptoms, clinical examinations, and sleep registrations (apnea-hypopnea index (AHI) ≥ 5/hour or respiratory event index (REI) ≥ 5/hour).11,12 Further details are available at the FinnGen website. Notably, no overlap was observed, given that samples of CIPs and OSA originated from distinct consortiums.
Genetic Instrumental Variables (IVs) Selection
To align with the MR assumptions illustrated in Figure 1, we meticulously selected single nucleotide polymorphisms (SNPs) strongly correlated with the exposure as IVs.
For forward MR, we identified independent genetic variants in CIPs that reached genome-wide significance (p 8), ie SNPs. However, the initial SNP selection using this criterion demonstrated limitations. Previous studies indicate the challenge of attaining the gold standard threshold of p 8 for SNP selection in certain non-disease-related human phenotypes, such as gut microbiota, metabolic products, and immunological characteristics. Thus, for present MR analysis, setting the threshold between 5×10⁻⁶ and 5×10⁻⁵ is feasible.18–22 However, for subsequent removal of linkage disequilibrium (LD), maintaining the most rigorous standards is essential, specifically (10,000-kilobase distance, r² 8, using a similar method (10,000-kilobase distance, r² 23 Additionally, palindrome SNPs were excluded due to their unique nature, wherein the allele on the positive strand matches that on the negative strand, rendering it challenging to determine the strand carrying the effect allele. Finally, for each SNP meeting the specified criteria, we computed its F-value and R² statistic, excluding SNPs with an F-value less than 10, as these are deemed weak IVs with inadequate correlation between genetic variation and exposure factors.24
Notes: EAF denotes the effect allele frequency of the exposure variable, N represents the sample size of the exposure variable, beta signifies the estimated genetic effect on the exposure variable, and SE (beta) corresponds to the standard error of the genetic effect.
Bidirectional Mendelian Randomization Study
This study employed summary-level data to investigate the causal relationships between CIPs and OSA. The causal relationships can be appraised through the method of inverse variance weighting (IVW). It stands as the most potent approach within Mendelian randomization analysis, possessing maximal statistical efficacy.23 Five primary MR analysis methods were employed, with the IVW method as the principal approach, supplemented by MR Egger, Weighted median, Simple mode, and Weighted mode.
The impact of exposure factors on outcomes was communicated through odds ratios (OR) accompanied by the corresponding 95% confidence intervals (CI). To enhance the presentation of results, we employed various visualization tools, including forest plots, funnel plots, and scatter plots. Ultimately, leveraging the P-values from the five MR methods and the OR values obtained from the IVW method, we constructed a circular heatmap. This visualization was created using the circlize (version 0.4.15), ComplexHeatmap (version 2.16.0), tidyverse (version 2.0.0), ggpubr (version 0.6.0) and ggplot2 (version 3.4.4) packages within the R software environment (version 4.3.1).
Sensitivity Analysis
We performed multiple sensitivity analyses using methodologies that incorporated distinct assumptions regarding horizontal pleiotropy, encompassing MR Egger and MR-pleiotropy residual sum and outlier (MR-PRESSO). MR Egger analysis was utilized to assess pleiotropy, with a nonzero intercept indicating potential bias in the IVW estimation.25 MR-PRESSO utilized a comprehensive test for identifying horizontal pleiotropy, and if necessary, potential pleiotropic outliers were corrected by their removal.26 Furthermore, we implemented outlier exclusion of anomalous SNPs using the “leave-one-out” method to ensure that individual SNP did not disproportionately impact the causal relationship between the exposure and outcome. For evaluating heterogeneity, Cochran Q tests were performed in both IVW and MR Egger methods. When the P-value was less than 0.05, indicating the presence of heterogeneity, the IVW random-effects model was employed to estimate SNP effects. Otherwise, the IVW fixed-effects model was presented as the result of the IVW method.
Statistical Analysis
Confronted with the challenge of multiple comparisons and drawing from insights gained in previous research,18,22 we applied Bonferroni correction to mitigate potential inflation of the statistical significance level across multiple tests. Specifically, Bonferroni correction involves dividing the significance level (typically 0.05) by the number of comparisons. As a result, associations with a P-value below 0.000549 (0.05/91) were deemed robust evidence of association, while results falling between 0.000549 and 0.05 were considered suggestive associations. Data analyses were executed using the MRPRESSO (version 1.0) and TwoSampleMR (version 0.5.6) packages in R software (version 4.3.1), reporting adheres to the STROBE-MR statement.27
Results
Causal Effects of CIPs on OSA
Considering the constrained range of genetic variation, the restricted number of SNPs, and their relatively modest effect sizes, we performed a MR analysis with a permissive P-value threshold of 5×10⁻⁶. Each SNP among the 91 studied CIPs exhibited an F-value exceeding 10, making a substantial weak instrument bias improbable. For additional details, refer to Supplementary Table 1. The 91 CIPs were classified into four categories: Interleukins, Chemokines, Growth factors, and Others. The positive findings obtained through the forward MR analysis using the IVW method are depicted in Figure 2, while the remaining negative results can be found in Supplementary Figure 1. Refer to Supplementary Table 2 for results of the five MR analysis methods on all 91 CIPs as exposures. MR Egger and MR-PRESSO analyses suggested the absence of horizontal pleiotropy (Pleiotropy_P and MR PRESSO_P were both greater than 0.05). In cases when the Cochran Q-test P-value was less than 0.05, indicating heterogeneity, the IVW results selected the random-effects model. For those CIPs without heterogeneity, the IVW results chose the fixed-effects model. The detailed results of the sensitivity analysis are provided in Supplementary Table 3.
As exposures of CIPs, we identified 12 CIPs associated with OSA. Among them, 5 were positively associated with OSA: IL-17C (OR, 1.090, 95% CI, 1.008–1.178, p = 0.0311), CCL25 (OR, 1.079, 95% CI, 1.000–1.164, p = 0.0493), FGF-5 (OR, 1.090, 95% CI, 1.041–1.142, p = 0.0003), CD5 (OR, 1.055, 95% CI, 1.001–1.113, p = 0.0477), TNFSF14 (OR, 1.092, 95% CI, 1.037–1.150, p = 0.0008). 7 CIPs were negatively associated with OSA: IL-20RA (OR, 0.877, 95% CI, 0.793–0.970, p = 0.0107), CCL19 (OR, 0.933, 95% CI, 0.879–0.991, p = 0.0237), MIP-1 alpha (OR, 0.906, 95% CI, 0.846–0.969, p = 0.0042), Flt3L (OR, 0.941, 95% CI, 0.905–0.978, p = 0.0019), CST5 (OR, 0.957, 95% CI, 0.919–0.996, p = 0.0320), OPG (OR, 0.850, 95% CI, 0.786–0.920, p = 0.0001), TRAIL (OR, 0.956, 95% CI, 0.925–0.987, p= 0.0063).
FGF-5 and OPG had P-values less than 0.000549, indicating a robust correlation with OSA, while the remaining 10 CIPs showed suggestive associations. Scatter plots for the 12 CIPs using the five MR methods are depicted in Figure 3. Corresponding forest plots, funnel plots, and “leave-one-out” plots can be found in, Supplementary Figures 2–4 respectively. Finally, the circular heatmap illustrating CIPs as the exposure and OSA as the outcome is presented as Panel A in Figure 4.
Causal Effects of OSA on CIPs
In the summary-level OSA data, we selected SNPs based on the threshold of p 8. After removing linkage disequilibrium and palindromic SNPs, seventeen significant SNPs were selected as IVs for OSA. The F-values for each SNP exceeded 10, indicating that the presence of a weak instrument bias is improbable to be substantial. For additional details, refer to Supplementary Table 4. Considering 91 CIPs as outcomes, categorized into Interleukins, Chemokines, Growth factors and Others, the positive outcomes from the MR analysis conducted in reverse using the IVW method are illustrated in Figure 2, whereas the additional negative results are available in Supplementary Figure 5. The results of five MR analysis methods for all 91 CIPs as outcomes are presented in Supplementary Table 5. Both MR Egger and MR-PRESSO analyses suggested the absence of horizontal pleiotropy (Pleiotropy_P and MR PRESSO_P were both greater than 0.05), and Cochran Q-test P-values were all greater than 0.05, indicating no heterogeneity. Therefore, the IVW results chose the fixed-effects model. The detailed results of the sensitivity analysis are provided in Supplementary Table 6.
As outcomes, we identified 5 CIPs associated with OSA. Among them, OSA was positively correlated with one CIP, IL-10RA (OR, 1.153, 95% CI, 1.001–1.327, p = 0.0478). Furthermore, OSA was negatively correlated with 4 CIPs, namely CCL28 (OR, 0.875, 95% CI, 0.774–0.988, p = 0.0317), DNER (OR, 0.874, 95% CI, 0.772–0.989, p = 0.0324), FGF-21 (OR, 0.846, 95% CI, 0.725–0.988, p = 0.0344), CSF-1 (OR, 0.842, 95% CI, 0.715–0.992, p = 0.0396).
The P-values for these 5 CIPs were all between 0.000549 and 0.05, indicating a suggestive association between OSA and these CIPs. Scatter plots for these 5 CIPs using the five MR methods are depicted in Figure 5. Corresponding forest plots, funnel plots, and “leave-one-out” plots can be found in, Supplementary Figures 6–8 respectively. Lastly, the circular heatmap depicting OSA as the exposure and CIPs as the outcome is displayed as Panel B in Figure 4.
Discussion
In our investigation, we performed a bidirectional MR analysis using the latest GWAS summary-level data to evaluate the causal associations between CIPs and OSA. Within this bidirectional MR analysis, we identified associations among the 91 CIPs. IL-17C, CCL25, FGF-5, CD5 and TNFSF14 were found to be associated with an increased risk of OSA, while IL-20RA, CCL19, CST5, Flt3L, MIP-1 alpha, OPG and TRAIL may reduce the risk of developing OSA. In contrast, our findings also suggested a causal association between OSA and elevated IL-10RA level, OSA may potentially lead to a decrease in CCL28, DNER, FGF-21 and CSF-1 levels. Additionally, we did not observe evidence supporting causal relationships between other CIPs and OSA, and there was no evidence of a reverse causal relationship between individual CIPs and OSA. In conclusion, our findings suggest that certain CIPs may act as precursors in the occurrence and development of OSA, while others are more likely to be downstream factors in the advancement of the condition.
Our findings substantiate the hypothesis that specific CIPs play a pivotal role in promoting the development of OSA. Notably, leveraging them as prognostic markers for OSA holds crucial significance. The intermittent hypoxia induced by OSA surpasses the extent and range of damage observed in conventional respiratory diseases characterized by sustained hypoxia. This hypoxic pattern, characterized by significant variability, bears similarities to ischemia-reperfusion injury. It forms the pathophysiological foundation for heightened sympathetic nervous system excitability, the emergence of systemic oxidative stress, and inflammatory reactions. As the disease progresses, the inflicted damage can extend to multiple bodily systems.28,29 Consequently, comprehending the pathways through which CIPs participate in the inflammatory response process holds paramount importance for prospective targeted interventions in the realm of inflammatory mechanisms.
Interleukins, primarily produced by white blood cells, play a pivotal role in maintaining the balance of the immune system, inflammation, and physiological processes such as cell signal transduction. Within the context of inflammatory responses, they actively participate in various inflammation pathways, serving indispensable functions in the body’s pathophysiological metabolism. Research indicates a significant elevation in serum levels of IL-35 and IL-37 in OSA patients, correlating with the severity of OSA.30 Studies by Vida Motamedi et al further affirmed the elevation of IL-6 concentrations in patients with moderate to severe OSA.31 Li-Pang Chuang and team’s research discovered an increase in the expression of monocyte IL-8 due to intermittent hypoxia.32
IL-17, primarily secreted by activated T lymphocytes, currently has six family members (IL-17A to IL-17F). IL-17C is mainly expressed in CD4+ T cells at inflammatory sites and is not expressed in most normal tissues. It is associated with increased production of TNF-α and IL-6 by macrophages.33 IL-17C fosters inflammation in an autocrine manner,34 eliciting epithelial inflammatory responses such as the expression of pro-inflammatory cytokines, chemokines, and antimicrobial peptides, enhancing immune barriers.35 Research has shown a close relationship between IL-17C and the inflammatory processes in chronic obstructive pulmonary disease (COPD).36 Our study found that an increase in IL-17C level increases the risk of OSA, and the specific mechanisms need further exploration. IL-10RA and IL-20RA are the α subunits of the IL-10 and IL-20 receptors, respectively. The former, as a downstream effector protein of OSA, is increased in expression in OSA patients, while the latter, as an upstream regulatory protein of OSA, may be a protective factor for OSA. Currently, there is no detailed study on the subunits of interleukin receptors, and further clinical research is needed for confirmation.
Chemokines play a guiding role in the migration of immune cells to infection and inflammation sites during the body’s inflammatory response. The C-C motif class chemokines, encompassing a minimum of 28 constituents (C-C motif chemokine ligands (CCLs) 1–28), transduce signals via 10 identified chemokine receptors (C-C motif chemokine receptors (CCRs) 1–10).37 CCL19 serves as a leukocyte chemoattractant, playing a pivotal role in modulating the migration and positioning of immune cells, including lymphocytes and dendritic cells.
CCR7 serves as the receptor for CCL19, and study suggests that the activation of the CCL19/CCR7 pathway in adipose tissue triggers inflammation. CCL19 derived from adipocytes inhibits AMPKα by activating ERK1/2, thereby causing impaired lipid metabolism and disruption of energy regulation.38 In a study employing a murine model of chronic hepatitis B (CHB), the upregulation of CCL19 expedited the elimination of HBV in the mouse liver and facilitated an augmentation of intrahepatic CD8 T cells.39 Our study found that elevated expression of CCL19 has a protective effect against OSA. CCL25’s receptor is CCR9, and research shows that CCL25 is highly expressed in the intestinal epithelium and thymus.40 Additionally, CCR9/CCL25 is implicated in diverse inflammatory conditions, such as rheumatoid arthritis, inflammatory bowel disease, and asthma, fostering inflammatory reactions.41–43 Our research also confirms a positive correlation between elevated expression of CCL25 and the risk of OSA. CCL28 is expressed by columnar epithelial cells in the intestines, lungs and salivary glands. Through interaction with its chemokine receptors CCR10 and CCR3, it participates in host immunity. Studies confirm its potent antimicrobial activity against a broad spectrum of microorganisms in mucosal immunity.44 Our study reveals that OSA can lead to a decrease in CCL28 level, which may reduce the anti-inflammatory capacity of OSA patients to some extent and may make them more susceptible to microbial invasion.
MIP-1 alpha belongs to the C-C subfamily of chemokines. It not only exhibits chemotactic effects on white blood cells, participating in inflammation, immune responses, and the anti-infection process, but it can also inhibit the proliferation of hematopoietic stem cells.45 Studies have found elevated level of MIP-1 alpha in the bone marrow of patients with multiple myeloma, and in severe bone disease patients, an increase in plasma has even been observed, indicating its osteoclast activity and often associated with a poor prognosis.46,47 Our study suggests that MIP-1 alpha is a protective factor in OSA. The signaling pathways it may be involved in OSA are not yet clear and require further validation.
The mammalian fibroblast growth factor (FGF) family consists of 22 proteins (FGF1-FGF23, with FGF15 yet to be discovered), primarily divided into two categories: secretory signaling proteins (secretory FGF) and intracellular non-signaling proteins (intracellular FGF). The former can signal to receptor tyrosine kinases, regulating fundamental cellular processes and playing a crucial role in injury and tissue repair.48 The latter, serving as auxiliary factors for voltage-gated sodium channels and other molecules, are essential regulators of neuron and cell excitability.49 FGFs can also be categorized into intracrine, paracrine, and endocrine classifications. FGF-5 is classified as a paracrine FGF, possessing a cleavable N-terminal signal peptide. It can mediate the biological responses of extracellular proteins by binding and activating cell surface tyrosine kinase FGFR.50 In contrast, FGF-21 falls under the category of endocrine FGFs, displaying a relatively lower affinity for heparin binding. This attribute endows it with endocrine functionality.51 FGF-21 is primarily expressed in the liver. Research indicates that fasting can increase the expression of hepatic FGF-21, and FGF-21 plays a crucial role in regulating lipolysis in white adipose tissue.52 Our study found that an elevated level of FGF-5 may increase the risk of OSA, while OSA can, to some extent, lead to a decrease in FGF-21 level.
DNER is a transmembrane protein specifically expressed in the central nervous system, carrying multiple epidermal growth factor-like repeat sequences. Abundant expression of DNER is present in the Purkinje cells of the cerebellum and the pyramidal cells of the hippocampus.53 The Notch signaling pathway is of fundamental importance in the development of the nervous system, and DNER mediates Notch signal transduction.54 Studies involving the knockout of the DNER gene in mice revealed that the knockout mice exhibited motor coordination deficits in experiments, and their cerebellar morphological development was significantly delayed, confirming the crucial role of DNER in cerebellar development.55 Our research indicates that OSA may lead to a decrease in DNER level, highlighting the necessity for further investigation into the coordination abilities of OSA patients in clinical practice.
The Fms-related tyrosine kinase 3 ligand (Flt3L) is characterized as a type I transmembrane protein. When it binds to the Flt3 receptor, it promotes the growth of progenitor cells in the bone marrow and blood, thereby enhancing hematopoiesis. Moreover, it is highly expressed with Flt3 in the majority of leukemia patients.56 Additionally, elevated level of Flt3L have been found in the serum of rheumatoid arthritis patients.57 Our study indicates a negative correlation between high level of Flt3L and OSA. This may be attributed to its ability to enhance hematopoiesis, leading to increased oxygen uptake by red blood cells and subsequently reducing the occurrence of intermittent hypoxemia. However, these are speculative hypotheses that require further validation.
CSF-1, as a growth factor, plays a pivotal role in regulating the development, proliferation, and differentiation of mononuclear macrophages. Through its binding with CSF-1R on the cell surface, it activates the phosphorylation of multiple intracellular tyrosine residues, initiating a series of signal cascades based on phosphotyrosine, and thereby participating in various cellular activities.58 Additionally, it also possesses a certain chemotactic effect on mononuclear macrophages. A study using a mouse model suggests that CSF-1 may play a role in the genesis of inflammatory pain.59 Nevertheless, this research specifically focuses on a mouse arthritis model and requires validation in more extensive disease cohorts. Our investigation indicates a potential reduction in CSF-1 level induced by OSA.
CD5, as a cell surface molecule, is affiliated with the scavenger receptor cysteine-rich (SRCR) superfamily, rich in cysteine. Studies have found that CD5 is a key regulatory factor in immune tolerance.60 In addition, it is involved in various signaling pathways of B lymphocytes, including extracellular signal-regulated kinases (ERK1/2), phosphatidylinositol 3-kinase (PI-3K), and calcineurin. Stimulation of these pathways results in the activation of transcription factors, including NFAT2 and STAT3, thereby participating in the production of IL-10, indirectly affecting the biological functions of B lymphocytes.61 Currently, research on CD5 is more in-depth in autoimmune diseases and leukemia. Our study found that an increase in CD5 may elevate the risk of OSA, suggesting that immune regulatory mechanisms may also be involved in the occurrence and development of OSA, but further clinical validation is needed.
CST5, also known as Cystatin D, is a cysteine protease inhibitor that regulates intracellular protein degradation and metabolic processes by inhibiting the activity of certain proteases.62 In a study on traumatic brain injury (TBI), early changes in inflammatory proteins induced by TBI were observed, and CST5 was identified as a potential biomarker for early differentiation of TBI severity.63 Another investigation on colorectal cancer cells found that Cystatin D can modulate the expression of specific genes, and its activity may contribute to a tumor-suppressive effect on colorectal cancer.64 The elevated level of CST5 discovered in this research may confer a protective effect against OSA, suggesting a novel avenue for future OSA treatment.
TNFSF14 and TRAIL, both members of the tumor necrosis factor (TNF) family, play distinct roles in immune regulation and apoptosis. TNFSF14 can trigger signal transduction pathways by binding to its receptor, Herpesvirus Entry Mediator (HVEM), thereby influencing the activation and regulation of immune cells. It has been shown to have a dual role during various viral infections, regulating T cell activation, proliferation, and differentiation, while also promoting inflammation progression and potentially leading to tissue damage.65 Elevated TNFSF14 level have been observed in patients with sepsis, irrespective of viral or bacterial origin, indicating its involvement in various inflammation-related diseases.66 TRAIL induces apoptosis in malignant cells by activating the apoptotic pathway within cells through binding to its receptor and recruiting the death-inducing signaling complex (DISC).67 Hence, investigating the regulatory mechanisms of the signaling pathways in which it is involved is of considerable significance in the realm of cancer therapeutics. In our study, both TNFSF14 and TRAIL are identified as upstream regulatory proteins of OSA, but their regulatory directions are not consistent. An increase in TNFSF14 level is associated with an increased risk of OSA, while a decrease in TRAIL level is linked to an increased risk of developing OSA. These findings suggest that their respective biological functions may contribute to the development or progression of OSA.
OPG, a secreted glycoprotein, belongs to the TNF receptor superfamily. It participates in blocking the differentiation process of osteoclasts and is alternatively recognized as osteoclastogenesis inhibitory factor (OCIF). Due to its inhibitory effect on osteoclasts, OPG has significant implications in the treatment of conditions such as osteoporosis. Furthermore, research findings suggest that heightened level of OPG is linked to an augmented risk of cardiovascular diseases.68 OPG has been proposed as a biomarker predicting future cardiovascular complications.69 In the context of OSA, our study suggests that high level of OPG may serve as a protective factor against OSA, potentially improving the stability of the upper airway and reducing the risk of upper airway collapse. It is crucial to emphasize that these findings are speculative, and the specific mechanisms underlying the association between OPG level and OSA need further investigation through clinical research for validation.
This is currently the first large-scale bidirectional MR study addressing the causal relationships between 91 CIPs and OSA. However, some limitations must be considered. Firstly, summary-level OSA data are derived from large-scale GWAS studies, and due to the lack of specific severity grading for OSA patients, subgroup analysis cannot be conducted. Secondly, as the study population consists of individuals of European descent, caution should be exercised when applying the conclusions to populations in other regions. Thirdly, further clinical studies are still needed to confirm our results, or clinical research may draw inspiration to further unveil OSA’s inflammatory response, facilitating the development of new diagnostic and therapeutic approaches to collectively promote clinical progress.
Conclusion
In this bidirectional MR study, we identified 12 upstream regulatory proteins and 5 downstream effect proteins associated with OSA. This may offer potential therapeutic targets for the inflammatory mechanisms of OSA.
Data Sharing Statement
The original contributions proposed in this study are located in the main text/supplementary materials. The GWAS data used has been appropriately cited. Further inquiries can be directed to the corresponding author.
Ethics Approval
The present study was strictly conducted in accordance with the Declaration of Helsinki and International Ethical Guidelines for Health-related Research Involving Humans. This study obtained approval from the Medical Ethics Committee of Tianjin Chest Hospital, with review opinion number 2024LW-007.
Acknowledgments
The authors wish to express gratitude to the researchers and participants involved in the original GWAS and FinnGen biobank for their efforts in collecting and managing extensive data resources, as well as those who actively participated in this study.
Author Contributions
All authors made a significant contribution to the work reported, whether that is in the conception, study design, execution, acquisition of data, analysis and interpretation, or in all these areas; took part in drafting, revising or critically reviewing the article; gave final approval of the version to be published; have agreed on the journal to which the article has been submitted; and agree to be accountable for all aspects of the work.
Funding
This work was funded by grants from National Clinical Key Specialty Construction Project, Tianjin Key Medical Discipline (Specialty) Construction Project (TJYXZDXK-049A).
Disclosure
The authors report no conflicts of interest in this work.
References
1. Lévy P, Kohler M, McNicholas WT, et al. Obstructive sleep apnoea syndrome. Nat Rev Dis Primers. 2015;1:15015. doi:10.1038/nrdp.2015.15
2. Benjafield AV, Ayas NT, Eastwood PR, et al. Estimation of the global prevalence and burden of obstructive sleep apnoea: a literature-based analysis. Lancet Respir Med. 2019;7(8):687–698. doi:10.1016/s2213-2600(19)30198-5
3. Franklin KA, Lindberg E. Obstructive sleep apnea is a common disorder in the population-a review on the epidemiology of sleep apnea. J Thorac Dis. 2015;7(8):1311–1322. doi:10.3978/j.issn.2072-1439.2015.06.11
4. Unnikrishnan D, Jun J, Polotsky V. Inflammation in sleep apnea: an update. Rev Endocr Metab Disord. 2015;16(1):25–34. doi:10.1007/s11154-014-9304-x
5. Ciccone MM, Scicchitano P, Zito A, et al. Correlation between inflammatory markers of atherosclerosis and carotid intima-media thickness in Obstructive Sleep Apnea. Molecules. 2014;19(2):1651–1662. doi:10.3390/molecules19021651
6. Unüvar Doğan F, Yosunkaya S, Kuzu Okur H, Can U. Relationships between Obstructive Sleep Apnea Syndrome, Continuous Positive Airway Pressure Treatment, and Inflammatory Cytokines. Sleep Disord. 2014;2014:518920. doi:10.1155/2014/518920
7. Stradling JR, Craig SE, Kohler M, et al. Markers of inflammation: data from the MOSAIC randomised trial of CPAP for minimally symptomatic OSA. Thorax. 2015;70(2):181–182. doi:10.1136/thoraxjnl-2014-205958
8. Pau MC, Zinellu A, Mangoni AA, et al. Evaluation of Inflammation and Oxidative Stress Markers in Patients with Obstructive Sleep Apnea (OSA). J Clin Med. 2023;12(12):3935. doi:10.3390/jcm12123935
9. Smith GD, Ebrahim S. ‘Mendelian randomization’: can genetic epidemiology contribute to understanding environmental determinants of disease? Int J Epidemiol. 2003;32(1):1–22. doi:10.1093/ije/dyg070
10. Hartwig FP, Davies NM, Hemani G, Davey Smith G. Two-sample Mendelian randomization: avoiding the downsides of a powerful, widely applicable but potentially fallible technique. Int J Epidemiol. 2016;45(6):1717–1726. doi:10.1093/ije/dyx028
11. Xu H, Liu F, Li Z, et al. Genome-Wide Association Study of Obstructive Sleep Apnea and Objective Sleep-related Traits Identifies Novel Risk Loci in Han Chinese Individuals. Am J Respir Crit Care Med. 2022;206(12):1534–1545. doi:10.1164/rccm.202109-2044OC
12. Strausz S, Ruotsalainen S, Ollila HM, et al. Genetic analysis of obstructive sleep apnoea discovers a strong association with cardiometabolic health. Eur Respir J. 2021;57(5):2003091. doi:10.1183/13993003.03091-2020
13. Yi M, Zhao W, Fei Q, et al. Causal analysis between altered levels of interleukins and obstructive sleep apnea. Front Immunol. 2022;13:888644. doi:10.3389/fimmu.2022.888644
14. Yi M, Zhao W, Tan Y, et al. The causal relationships between obstructive sleep apnea and elevated CRP and TNF-α protein levels. Ann Med. 2022;54(1):1578–1589. doi:10.1080/07853890.2022.2081873
15. Emdin CA, Khera AV, Kathiresan S. Mendelian Randomization. JAMA. 2017;318(19):1925–1926. doi:10.1001/jama.2017.17219
16. Zhao JH, Stacey D, Eriksson N, et al. Genetics of circulating inflammatory proteins identifies drivers of immune-mediated disease risk and therapeutic targets. Nat Immunol. 2023;24(9):1540–1551. doi:10.1038/s41590-023-01588-w
17. Kurki MI, Karjalainen J, Palta P, et al. FinnGen provides genetic insights from a well-phenotyped isolated population. Nature. 2023;613(7944):508–518. doi:10.1038/s41586-022-05473-8
18. Zhang J, Chen X, Zhu Y, Wan S, Hu S, Yang Y. Investigating the Causal Relationship Between Sleep Behaviors and Primary Open-Angle Glaucoma: A Bidirectional Two-Sample Mendelian Randomization Study. Nat Sci Sleep. 2024;16:143–153. doi:10.2147/NSS.S439274
19. Li P, Wang H, Guo L, et al. Association between gut microbiota and preeclampsia-eclampsia: a two-sample Mendelian randomization study. BMC Med. 2022;20(1):443. doi:10.1186/s12916-022-02657-x
20. Gu Y, Jin Q, Hu J, et al. Causality of genetically determined metabolites and metabolic pathways on osteoarthritis: a two-sample mendelian randomization study. J Transl Med. 2023;21(1):357. doi:10.1186/s12967-023-04165-9
21. Wang C, Zhu D, Zhang D, et al. Causal role of immune cells in schizophrenia: Mendelian randomization (MR) study. BMC Psychiatry. 2023;23(1):590. doi:10.1186/s12888-023-05081-4
22. Xiang M, Wang Y, Gao Z, et al. Exploring causal correlations between inflammatory cytokines and systemic lupus erythematosus: A Mendelian randomization. Front Immunol. 2023;13:985729. doi:10.3389/fimmu.2022.985729
23. Burgess S, Butterworth A, Thompson SG. Mendelian randomization analysis with multiple genetic variants using summarized data. Genet Epidemiol. 2013;37(7):658–665. doi:10.1002/gepi.21758
24. Papadimitriou N, Dimou N, Tsilidis KK, et al. Physical activity and risks of breast and colorectal cancer: a Mendelian randomisation analysis. Nat Commun. 2020;11(1):597. doi:10.1038/s41467-020-14389-8
25. Bowden J, Davey Smith G, Burgess S. Mendelian randomization with invalid instruments: effect estimation and bias detection through Egger regression. Int J Epidemiol. 2015;44(2):512–525. doi:10.1093/ije/dyv080
26. Verbanck M, Chen CY, Neale B, Do R. Detection of widespread horizontal pleiotropy in causal relationships inferred from Mendelian randomization between complex traits and diseases. Nat Genet. 2018;50(5):693–698. doi:10.1038/s41588-018-0099-7
27. Skrivankova VW, Richmond RC, Woolf BAR, et al. Strengthening the Reporting of Observational Studies in Epidemiology Using Mendelian Randomization: The STROBE-MR Statement. JAMA. 2021;326(16):1614–1621. doi:10.1001/jama.2021.18236
28. Javaheri S, Barbe F, Campos-Rodriguez F, et al. Sleep Apnea: Types, Mechanisms, and Clinical Cardiovascular Consequences. J Am Coll Cardiol. 2017;69(7):841–858. doi:10.1016/j.jacc.2016.11.069
29. Mateika JH, Syed Z. Intermittent hypoxia, respiratory plasticity and sleep apnea in humans: present knowledge and future investigations. Respir Physiol Neurobiol. 2013;188(3):289–300. doi:10.1016/j.resp.2013.04.010
30. Chen B, Liu YN, Ji L, et al. Elevated levels of interleukin-35 and interleukin-37 in adult patients with obstructive sleep apnea. J Clin Lab Anal. 2021;35(6):e23790. doi:10.1002/jcla.23790
31. Motamedi V, Kanefsky R, Matsangas P, et al. Elevated tau and interleukin-6 concentrations in adults with obstructive sleep apnea. Sleep Med. 2018;43:71–76. doi:10.1016/j.sleep.2017.11.1121
32. Chuang LP, Wu HP, Lee LA, et al. Elevated Monocytic Interleukin-8 Expression under Intermittent Hypoxia Condition and in Obstructive Sleep Apnea Patients. Int J Mol Sci. 2021;22(21):11396. doi:10.3390/ijms222111396
33. Yamaguchi Y, Fujio K, Shoda H, et al. IL-17B and IL-17C are associated with TNF-alpha production and contribute to the exacerbation of inflammatory arthritis. J Immunol. 2007;179(10):7128–7136. doi:10.4049/jimmunol.179.10.7128
34. Ramirez-Carrozzi V, Sambandam A, Luis E, et al. IL-17C regulates the innate immune function of epithelial cells in an autocrine manner. Nat Immunol. 2011;12(12):1159–1166. doi:10.1038/ni.2156
35. Nies JF, Panzer U. IL-17C/IL-17RE: Emergence of a Unique Axis in TH17 Biology. Front Immunol. 2020;11:341. doi:10.3389/fimmu.2020.00341
36. Vella G, Ritzmann F, Wolf L, et al. IL-17C contributes to NTHi-induced inflammation and lung damage in experimental COPD and is present in sputum during acute exacerbations. PLoS One. 2021;16(1):e0243484. doi:10.1371/journal.pone.0243484
37. White GE, Iqbal AJ, Greaves DR. CC chemokine receptors and chronic inflammation–therapeutic opportunities and pharmacological challenges. Pharmacol Rev. 2013;65(1):47–89. doi:10.1124/pr.111.005074
38. Hayashi M, Iwashita M, Nishimura Y, et al. Adipose-specific C-C motif chemokine ligand (CCL) 19 overexpression drives the mice to both insulin resistance and weight gain. BMJ Open Diabetes Res Care. 2021;9(1):e001871. doi:10.1136/bmjdrc-2020-001871
39. Yan Y, Zhao W, Liu W, et al. CCL19 enhances CD8+ T-cell responses and accelerates HBV clearance. J Gastroenterol. 2021;56(8):769–785. doi:10.1007/s00535-021-01799-8
40. Wu X, Sun M, Yang Z, et al. The Roles of CCR9/CCL25 in Inflammation and Inflammation-Associated Diseases. Front Cell Dev Biol. 2021;9:686548. doi:10.3389/fcell.2021.686548
41. Yokoyama W, Kohsaka H, Kaneko K, et al. Abrogation of CC chemokine receptor 9 ameliorates collagen-induced arthritis of mice. Arthritis Res Ther. 2014;16(5):445. doi:10.1186/s13075-014-0445-9
42. Wurbel MA, McIntire MG, Dwyer P, Fiebiger E. CCL25/CCR9 interactions regulate large intestinal inflammation in a murine model of acute colitis. PLoS One. 2011;6(1):e16442. doi:10.1371/journal.pone.0016442
43. López-Pacheco C, Soldevila G, Du Pont G, Hernández-Pando R, García-Zepeda EA. CCR9 Is a Key Regulator of Early Phases of Allergic Airway Inflammation. Mediators Inflamm. 2016;2016:3635809. doi:10.1155/2016/3635809
44. Hieshima K, Ohtani H, Shibano M, et al. CCL28 has dual roles in mucosal immunity as a chemokine with broad-spectrum antimicrobial activity. J Immunol. 2003;170(3):1452–1461. doi:10.4049/jimmunol.170.3.1452
45. Cook DN. The role of MIP-1 alpha in inflammation and hematopoiesis. J Leukoc Biol. 1996;59(1):61–66. doi:10.1002/jlb.59.1.61
46. Terpos E, Politou M, Viniou N, Rahemtulla A. Significance of macrophage inflammatory protein-1 alpha (MIP-1alpha) in multiple myeloma. Leuk Lymphoma. 2005;46(12):1699–1707. doi:10.1080/10428190500175049
47. Hata H. Bone lesions and macrophage inflammatory protein-1 alpha (MIP-1a) in human multiple myeloma. Leuk Lymphoma. 2005;46(7):967–972. doi:10.1080/10428190500066636
48. Müller AK, Meyer M, Werner S. The roles of receptor tyrosine kinases and their ligands in the wound repair process. Semin Cell Dev Biol. 2012;23(9):963–970. doi:10.1016/j.semcdb.2012.09.015
49. Ornitz DM, Itoh N. The Fibroblast Growth Factor signaling pathway. Wiley Interdiscip Rev Dev Biol. 2015;4(3):215–266. doi:10.1002/wdev.176
50. Itoh N, Ornitz DM. Fibroblast growth factors: from molecular evolution to roles in development, metabolism and disease. J Biochem. 2011;149(2):121–130. doi:10.1093/jb/mvq121
51. Goetz R, Beenken A, Ibrahimi OA, et al. Molecular insights into the klotho-dependent, endocrine mode of action of fibroblast growth factor 19 subfamily members. Mol Cell Biol. 2007;27(9):3417–3428. doi:10.1128/mcb.02249-06
52. Hotta Y, Nakamura H, Konishi M, et al. Fibroblast growth factor 21 regulates lipolysis in white adipose tissue but is not required for ketogenesis and triglyceride clearance in liver. Endocrinology. 2009;150(10):4625–4633. doi:10.1210/en.2009-0119
53. Eiraku M, Hirata Y, Takeshima H, Hirano T, Kengaku M. Delta/notch-like epidermal growth factor (EGF)-related receptor, a novel EGF-like repeat-containing protein targeted to dendrites of developing and adult central nervous system neurons. J Biol Chem. 2002;277(28):25400–25407. doi:10.1074/jbc.M110793200
54. Eiraku M, Tohgo A, Ono K, et al. DNER acts as a neuron-specific Notch ligand during Bergmann glial development. Nat Neurosci. 2005;8(7):873–880. doi:10.1038/nn1492
55. Tohgo A, Eiraku M, Miyazaki T, et al. Impaired cerebellar functions in mutant mice lacking DNER. Mol Cell Neurosci. 2006;31(2):326–333. doi:10.1016/j.mcn.2005.10.003
56. Stirewalt DL, Radich JP. The role of FLT3 in haematopoietic malignancies. Nat Rev Cancer. 2003;3(9):650–665. doi:10.1038/nrc1169
57. Ramos MI, Perez SG, Aarrass S, et al. FMS-related tyrosine kinase 3 ligand (Flt3L)/CD135 axis in rheumatoid arthritis. Arthritis Res Ther. 2013;15(6):R209. doi:10.1186/ar4403
58. Mouchemore KA, Pixley FJ. CSF-1 signaling in macrophages: pleiotrophy through phosphotyrosine-based signaling pathways. Crit Rev Clin Lab Sci. 2012;49(2):49–61. doi:10.3109/10408363.2012.666845
59. Saleh R, Lee MC, Khiew SH, et al. CSF-1 in Inflammatory and Arthritic Pain Development. J Immunol. 2018;201(7):2042–2053. doi:10.4049/jimmunol.1800665
60. Raman C. CD5, an important regulator of lymphocyte selection and immune tolerance. Immunol Res. 2002;26(1–3):255–263. doi:10.1385/IR:26:1-3:255
61. Mageed RA, Garaud S, Taher TE, et al. CD5 expression promotes multiple intracellular signaling pathways in B lymphocyte. Autoimmun Rev. 2012;11(11):795–798. doi:10.1016/j.autrev.2012.02.007
62. Turk V, Stoka V, Vasiljeva O, et al. Cysteine cathepsins: from structure, function and regulation to new frontiers. Biochim Biophys Acta. 2012;1824(1):68–88. doi:10.1016/j.bbapap.2011.10.002
63. Hill LJ, Di Pietro V, Hazeldine J, et al. Cystatin D (CST5): An ultra-early inflammatory biomarker of traumatic brain injury. Sci Rep. 2017;7(1):5002. doi:10.1038/s41598-017-04722-5
64. Ferrer-Mayorga G, Alvarez-Díaz S, Valle N, et al. Cystatin D locates in the nucleus at sites of active transcription and modulates gene and protein expression. J Biol Chem. 2015;290(44):26533–26548. doi:10.1074/jbc.M115.660175
65. Hou Y, Wang Y, Chen J, Chen C. Dual Roles of Tumor Necrosis Factor Superfamily 14 in Antiviral Immunity. Viral Immunol. 2022;35(9):579–585. doi:10.1089/vim.2022.0070
66. Qu HQ, Snyder J, Connolly J, et al. Circulating LIGHT (TNFSF14) and Interleukin-18 Levels in Sepsis-Induced Multi-Organ Injuries. Biomedicines. 2022;10(2)264. doi:10.3390/biomedicines10020264
67. von Karstedt S, Montinaro A, Walczak H. Exploring the TRAILs less travelled: TRAIL in cancer biology and therapy. Nat Rev Cancer. 2017;17(6):352–366. doi:10.1038/nrc.2017.28
68. Kiechl S, Schett G, Wenning G, et al. Osteoprotegerin is a risk factor for progressive atherosclerosis and cardiovascular disease. Circulation. 2004;109(18):2175–2180. doi:10.1161/01.CIR.0000127957.43874.BB
69. Vik A, Mathiesen EB, Brox J, et al. Serum osteoprotegerin is a predictor for incident cardiovascular disease and mortality in a general population: the Tromsø Study. J Thromb Haemost. 2011;9(4):638–644. doi:10.1111/j.1538-7836.2011.04222.x