Fitness
Cornus officinalis & effectiveness of LWDHD in treating IVDD | JIR
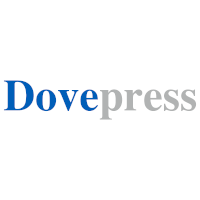
Introduction
Low back pain (LBP) is a prevalent clinical condition affecting individuals’ quality of life and yielding significant social and economic burdens. The prevalence of LBP has escalated from 377.5 million in 1990 to 577 million in 2017.1 This condition predominantly stems from intervertebral disc (IVD) degeneration (IVDD), impacting 26~42% of LBP patients.2,3 Existing therapeutic approaches encompass pharmaceutical, exercise, and surgical interventions,4 focusing on pain alleviation rather than halting the degeneration process, prompting a critical need for effective treatment modalities.
Liuwei Dihuang Decoction (LWDHD), a classic Traditional Chinese Medicine (TCM) prescription derived from the “Jingyue Quanshu”, consisting of 6 herbs, including Rehmannia glutinosa Libosch (Shu Di Huang), Dioscorea opposite Thunb (Shan Yao), Cornus officinalis Sieb. et Zucc (Shan Zhu Yu, CO), Paeonia suffruticosa Andr (Dan Pi), Alisma orientate (Sam.) Juzep (Ze Xie), Poria cocos (Schw.) Wolf (Fu Ling), has showcased significant efficacy in treating various kidney yin deficiency-induced diseases, including LBP.5,6 Our recent studies have underscored the therapeutic potential of LWDHD, including its component CO-derived chemical constituent morroniside, in improving lumbar spine instability (LSI) surgery-induced IVDD progression.7,8 Further investigations have demonstrated that loganin and morroniside, both chemical constituents sourced from CO, effectively delay joint degeneration by inhibiting chondrocytes pyroptosis,9,10 suggesting anti-inflammatory and anti-pyroptosis properties of CO in treating degenerative osteoarthropathies. In parallel, numerous findings from other researchers also demonstrate varied biological activities of CO and its bioactive components, including hypoglycemic, neuroprotective, heart-protective, hepatoprotective, nephroprotective, testis-protective activities, through anti-inflammatory, antioxidant activity, and anti-apoptosis mechanisms.11,12 Based on the above findings, we speculate that adjusting the dosage of CO in LWDHD may further enhance the therapeutic impact on IVDD. Nevertheless, uncertainties persist regarding the ideal CO dosage in the original LWDHD formulation and its potential mechanism for effectively treating IVDD.
The mechanisms of IVDD are complex, involving oxidative stress imbalances, reduction of nucleus pulposus (NP) cells, inflammation, and extracellular matrix (ECM) degradation.13 Notably, inflammatory elements such as Interleukin-1β (IL-1β) have been recognized as pivotal cytokine mediators involved in the pathological alterations of IVDD and LBP, which could intensify the inflammatory response, reduce ECM content by increasing matrix degradase such as matrix metalloproteinase 13 (MMP13), impair cell viability by promoting apoptosis and inhibiting proliferation, and foster neoinnervation.14,15
Pyroptosis is a newly discovered inflammatory programmed cell death process mediated by inflammasomes. Extensive works have highlighted the significance of IL-1β in IVDD, while the involvement of CASPASE1-mediated inflammasome in promoting various joint compartments has gained considerable attention. Both in vitro and in vivo experiments conducted by our team and other researchers have consistently demonstrated that aberrant oxidative stress-induced dysfunction of nuclear factor E2-related factor 2 (NRF2)/Kelch-like ECH-associated protein 1 (KEAP1) signaling can activate CASPASE1-mediated pyroptosis of NP cell, thereby triggering inflammation and exacerbating IVDD pathogenesis.13,16–18 Therefore, targeting NP cell pyroptosis and sensory innervation might offer potential benefits for slowing IVDD progression and relieving LBP.
This study aims to optimize the formulation through a comparative analysis of the therapeutic effects of varying doses of CO within LWDHD (1-, or 5- or 10-time dosage of original LWDHD formulation). To achieve this goal, LSI surgery-induced IVDD mice were treated with the original LWDHD formulation (referred to as 1 × CO or LWDHD thereafter), or a modified formulation of LWDHD with a 5- or 10-fold increase in the dosage of CO compared to the original formulation (referred to as 5 × CO and 10 × CO, respectively). Our findings will illuminate which dosage of CO within LWDHD yields the most optimal therapeutic efficacy for IVDD treatment.
Materials and Methods
Animals
Eight-week-old male C57BL/6J mice were provided by the animal experiments center of Zhejiang Chinese Medical University (Grade SPF, SCXK (Shanghai): 2017-0005). These mice were housed in a specific pathogen-free animal care facility under controlled conditions of 23 ± 2°C and a 12-h light/dark cycle. All animal experiments were obedient to the ARRIVE guidelines and conducted in accordance with the UK Animals (Scientific Procedures) Act, 1986 and related guidelines, EU Directive 2010/63/EU for animal experiments.19 They were given unrestricted access to water and lab chow. All animal experiments followed the National Institutes of Health Guidelines for the Care and Use of Laboratory Animals. All procedures involving the mice were approved by the Committee on the Ethics of Animal Experiments of Zhejiang Chinese Medical University (2020-KL-159-02).
Chemicals and Reagents
The dosage information of modified LWDHD formulations is presented in Table 1. Each set of medicinal materials was soaked overnight in a large beaker and then decocted in a stainless-steel pot with 2.5 L distilled water. The mixture was boiled down to 1.0 L and subsequently filtered to remove any herb residues. The resulting liquid medicine was then subpacked into 50 mL tubes and stored at –20°C. Prior to use, LWDHD was thawed in the refrigerator at 4°C and allowed to return to room temperature for 1–2 hours before gavage administration.
Table 1 Dosage of Modified LWDHD Formulations |
Primary antibody against AGGRECAN was from Abcam (Cambridge, MA, USA). Primary antibodies against Collagen 2 (COL2), MMP13, Ki-67, Gasdermin D (GSDMD), and IL-1β were purchased from Ruiying Biological (Jiangsu, China). Primary antibodies against Tyrosine kinase A (TrkA), and NRF2 (S40) were obtained from HuaBio (Hangzhou, China). Primary antibody against CASPASE1 was obtained from ProteinTech Group (Chicago, IL, USA). Primary antibody against KEAP1 was acquired from ImmunoWay Biotechnology Company (Jiangsu, China). Primary antibodies against phospho-I-κB (p-I-κB) and phospho-P65 (p-P65) were provided by Cell Signaling Technology (Beverly, MA, USA). Fluorescent secondary antibodies were supplied by Sungene Biotech Co. (Tianjin, China). Unless specified, all chemicals were from Sigma-Aldrich (St. Louis, MO).
Experimental Design
A total of 90 eight-week-old mice were distributed randomly and equally into five groups (n = 18): Sham group, Vehicle group, LWDHD group, 5 × CO group, and 10 × CO group. All mice, with the exception of those in the Sham group, underwent LSI surgery-induced IVDD modeling, as we previously described.8,16
The mice are anesthetized with isoflurane (3% isoflurane for induction, 2% isoflurane for maintenance). Following shearing and cleaning of the back, the back skin was disinfected with Iodophor and then longitudinally incised along the spine direction, the fascia was passively stripped, the erector spinal muscle of the mice was exposed, and the erector spinal muscles on both sides were passively separated axially (cutting off part of the erector spinal muscle if necessary). Subsequently, the lumbar spinous process of the mice was completely exposed, and the spinous process of L3 ~ L5 segments was completely excised. The supraspinous and interspinous ligaments were removed, followed by disinfection with Iodophor. Subsequently, a check was made for internal bleeding and peritoneum integrity. The muscles, fascia, and skin were sutured layer by layer, and gentamicin hydrochloride was applied to the wound to prevent infection.
Three days after LSI surgery, mice in the LWDHD group, 5 × CO group, and 10 × CO group were orally administered with the corresponding LWDHD (0.1 mL/20 g body weight) once a day for 8 consecutive weeks. The other two groups of mice received an equivalent dosage of normal saline. Following 1, 2, and 8 weeks post LSI surgery, 6 mice in each group were euthanized, and the lumbar vertebrae were collected for further analysis.
HPLC Assay of Morroniside, Loganin, and Paeoniflorin in Mice Plasma
The plasma of IVDD mice was collected following 1 and 8 weeks of LWDHD administration. And 100 μL of plasma from each mouse was combined with four times the volume of acetonitrile, vortex-mixed for 2 min, and centrifuged at 14000 rpm for 15 min. The resulting supernatant was dried using nitrogen blowing, and the residue was reconstituted with 200 μL of the initial mobile phase. After vortex-mixing for 30 seconds and centrifuging at 14000 rpm for 20 min, the supernatant was collected and injected for HPLC analysis.
Chromatographic conditions involved utilizing the Waters Xevo TQD triple quadrupole mass spectrometer, Waters Acquity ultrahigh performance liquid chromatograph, and the Waters ACQUITY UPLC C18 column (2.1100 mm, 1.7 m). The mobile phases included (A) 0.1% formic acid in water and (B) acetonitrile. The gradient elution occurred as follows: 0–6 min, 10–15% B; 6–10 min, 15–90% B; 10–10.1 min, 90–10% B; 10.1–12 min, 10% B. A flow rate of 0.3 mL/min, a column temperature of 30°C, and an injection volume of 3 μL were employed.
For mass spectrometric conditions, an electrospray ionization (ESI) source, negative ion detection, and multiple reaction detection modes (MRM) were used. The capillary voltage was set to 3.4 kV, the ion source temperature to 550°C, the degassing flow rate to 800 L/h, and the cone body flow rate to 50 L/h.
Functional Test
Rearing frequency analysis: The rearing frequency analysis involves placing the mouse in a 1 L beaker. Once the mouse is acclimated to its surroundings, the frequency of upright standing occurrences within one minute is recorded using a camera.
Von-Frey test: Allow the mice to acclimate to the environment for approximately 30 min until they exhibit minimal movement (no longer actively exploring). The middle part of the sole of the hind limb was gently stimulated with a Von-Frey fiber to bend the fiber for several seconds, and the mouse’s foot contraction reaction was observed. Rapid foot contraction reaction due to this stimulation is considered a positive response; care should be taken to differentiate this from a reaction caused by physical activity. If there are three positive reactions in five consecutive stimuli, it is marked as “×”; otherwise, it is marked as “O”. If a reaction occurs, the pressure is reduced to the previous level; if not, it is increased to the next level, and this operation is repeated (a total of six rounds). The pressure value associated with “×” (the pressure value corresponding to the last used fiber) was recorded for subsequent data analysis.
Open field test: The open field test device comprises an open field reaction box and an automatic data acquisition and processing system. The box measures 30 cm in height and 72 cm in length at the bottom, with the inner walls painted black. A digital camera is situated 2 meters above, with a field of vision covering the entire open field. The experiment is carried out in a quiet environment. Mice are placed in the center of the bottom inside the box, and simultaneous video recording and time tracking are initiated. Observation occurs over a 5-minute period. Following this, the inner walls and bottom of the box are cleaned, mice are replaced, and the test continues. Data are collected on total movement distance, central motion time, and distance relative to the center, for subsequent data processing.
HE Staining, Immunohistochemical (IHC), and Immunofluorescence (IF) Analyses
After fixation in 4% paraformaldehyde for 3 days, the IVD tissues were decalcified with 14% EDTA solution (pH = 7.4) for about 28 days, dehydrated in alcohol, embedded in paraffin, and sliced into 5 μm sections. The hematoxylin and eosin (HE) staining of the sections was carried out as we previously described.8,16 For IHC and IF analysis, the sections underwent thermal repair in sodium citrate buffer, followed by incubation with primary antibodies at specified dilutions (AGGRECAN (1:500 dilution), COL2 (1:300 dilution), MMP13 (1:300 dilution), Ki-67 (1:500 dilution), TrkA (1:500 dilution), CASPASE1 (1:500 dilution), GSDMD (1:500 dilution), IL-1β (1:500 dilution), NRF2 (S40) (1:500 dilution), KEAP1 (1:500 dilution), p-I-κB (1:500 dilution), and p-P65 (1:500 dilution)) at 4°C overnight, respectively. Negative control sections were incubated with nonspecific IgG. For IHC staining, the polymer-HRP labeled secondary antibody was added for 30 min the following day. The IHC reaction was visualized using 0.05% diaminobenzidine (ZSGB-BIO, Beijing, China) followed by counterstaining with hematoxylin. For IF analysis, the slides were incubated with fluorescence-conjugated secondary antibody for 30 min in the dark, and then DAPI staining was utilized to estimate the total cell number. Quantitative histomorphometric analysis was performed in a blinded manner using Image-Pro Plus Software version 6.0 (Media Cybernetics Inc., Rockville, Maryland, USA).
TUNEL Assay
The TUNEL assay to detect DNA breaks was performed using a TUNEL Bright Green Apoptosis Detection Kit (Vazyme Biotech; Nanjing, China), according to the manufacturer’s instruction. Negative controls were subjected to incubation in a TdT free-enzyme solution. The number of positive cells was quantified in three randomly selected fields of view using three sections from each sample. DAPI staining was utilized to estimate the total cell number.
Statistical Analysis
All numerical data are presented as mean ± standard error of mean (SEM). The statistical analysis was performed using GraphPad Prism 8 statistics software (San Diego, CA, USA). The differences between multiple groups were determined by two-way or one-way analysis of variance analysis (ANOVA) with Tukey’s multiple comparison test. p
Results
LWDHD Formulations Improve Lumbar Functional Damage in IVDD Mice
To investigate the effects of these 3 formulations on IVDD progression, mice subjected to LSI surgery received LWDHD, or 5 × CO or 10 × CO via intragastric administration for 8 weeks. The potential bioavailability of the formulations was assessed by determining the plasma concentrations of 3 quality control indexes of LWDHD, including morroniside, loganin, and paeoniflorin (mainly sourced from Dioscorea opposite Thunb), using HPLC after 1 and 8 weeks. Compared with original LWDHD, 8 weeks’ treatment with 5 × CO or 10 × CO treatment resulted in 2.4 folds and 2.2 folds increase of loganin, with a nonsignificant dose-dependent rise of morroniside. Conversely, no significant differences were observed in the concentrations of paeoniflorin (Figure S1).
To compare the pharmacological effect of these formulations on the lumbar function of IVDD mice, behavioral assessments, including rearing frequency analysis, Von-Frey test, and open field test, were carried out. IVDD mice exhibited a significant reduction in rearing frequency, while LWDHD and 5 × CO, but not 10 × CO, restored it to a level comparable to that of Sham mice (Figure 1A). Moreover, the Von-Frey test and open field test Results revealed that 5 × CO and 10 × CO, but not the original LWDHD, significantly reduced that central motion time, and no significant differences were observed in other indexes (Figure 1B and C), indicating a reduction in anxiety levels in IVDD mice (Figure 1C).
LWDHD Formulations Attenuate Structure Impairment and ECM Degradation in IVDs of IVDD Mice
To evaluate the impact of these LWDHD formulations on the structure and ECM composition of IVDs of IVDD mice, histomorphological alterations in L4-L5 IVDs were examined using HE staining. IVDD mice exhibited ruptured IVDs with flattened NP, fissures and folds in annulus fibrosus, and abnormal bone formation in endplates, and LWDHD and 5 × CO significantly reversed these phenotypes (Figure 2A). The degeneration of IVD was further assessed by the histological score system established by Norcross et al,20 and the results showed that administration with LWDHD and 5 × CO, but not 10 × CO, restored histological score (Figure 2B). Subsequently, the expressions of ECM such as AGGRECAN and COL2, and degradase MMP13 were analyzed using IHC analysis. The results showed that all LWDHD formulations significantly alleviate the decrease in AGGRECAN and COL2 and increase in MMP13 in IVDD mice, except for the lack of significant reversal of COL2 in IVDD mice treated with LWDHD (Figures 2C, D–H). Interestingly, 10 × CO treatment caused the most substantial restoration in AGGRECAN, while both the 5 × CO and 10 × CO formulations produced a comparable elevation in COL2. Conversely, the LWDHD led to the most pronounced reduction of MMP13.
LWDHD Formulations Mitigate NP Cell Viability and Invasion of Sensory Nerves in IVDD Mice
To assess whether modified formulations of LWDHD could affect NP cell viability and innervation in IVDD mice, the apoptosis and proliferation status of NP cells were determined using TUNEL assay and IF assay of Ki-67, a specific marker for proliferation. TUNEL results showed that 1 × CO and 10 × CO, but not 5 × CO, resulted in a significant reduction in TUNEL-positive cells in IVDs of IVDD mice (Figure 3A and B). Consistent with TUNEL results, IF results of Ki-67 showed that 1 × CO and 10 × CO, but not 5 × CO, led to a significant upregulation in Ki-67 expression of IVDs of IVDD mice (Figure 3C and D).
It has been well established that sensory nerve ingrowth into IVD was triggered by nerve growth factor/high-affinity TrkA signaling.21 To identify whether modified formulations of LWDHD could affect sensory nerve ingrowth, the expression of TrkA was assessed using IF analysis. The results showed that a noticeable increase in positive TrkA expression was observed in the NP of IVDD mice, and all 3 LWDHD formulations treatment decreased the increased expression of TrkA in IVDs of IVDD mice, with the 5 × CO-treated mice demonstrating the most pronounced inhibitory effect (Figure 3E and F). All these findings suggest that the efficacy of LWDHD with different dosages of CO in treating IVDD varies slightly.
LWDHD Formulations Inhibit NP Pyroptosis in IVDD Mice
In a previous study, we demonstrated that activated pyroptosis of NP cells is involved in the progression of IVDD.16 To analyze whether 3 LWDHD formulations could affect NP pyroptosis, the expression of key proteins in pyroptosis, including CASPASE1, GSDMD, and IL-1β was determined. Consistent with our previous results, we found a marked elevation in CASPASE1, GSDMD, and IL-1β expression within the NP tissues of IVDD mice, and all these formulations significantly reduced these elevations compared with IVDD model mice, except for a higher expression of GSDMD was noted in 10 × CO group compared to all other groups. Notably, among the LWDHD formulations, the 5 × CO treatment demonstrated superior efficacy in reducing the expression of CASPASE1, GSDMD, and IL-1β. Moreover, when compared with the 5 × CO group, 10 × CO treatment led to a slight increase in CASPASE1 and IL-1β, indicating a potential counterproductive effect of CO overdose (Figure 4A–F). These data suggest that the 5 × CO treatment is more effective in inhibiting NP pyroptosis.
LWDHD Formulations Activate NRF2/KEAP1 Pathway in NP Tissues
To elucidate the possible upstream mechanism through which LWDHD delays the progression of IVDD, we examined the activity of NRF2/KEAP1 pathway and downstream NF-κB pathway by analyzing the expression of antioxidant response protein NRF2 and its negative feedback protein KEAP1, as well as the p-I-κB and p-P65 using IF analysis. As shown in Figure 5, we found that LSI surgery resulted in almost no expression of NRF2 (S40) in IVDD mice, and 1.4-fold, 3.4-fold, and 8.7-fold increases of KEAP1, p-I-κB, and p-p65. Notably, 3 LWDHD formulation significantly reversed the decrease of NRF2 (S40) in a dose-dependent manner, while 1 × CO and 5 × CO significantly reduced the increased expression of KEAP1 to the level of Sham mice, except that 10 × CO treatment resulted in a higher KEAP1 than all other groups (2.3-fold of Sham mice). Intriguingly, all 3 LWDHD formulations could significantly reduce the elevated expression of p-P65, with 1 × CO and 10 × CO demonstrating significantly more inhibitory effects on the increase of p-P65 expression in IVDD mice than 5 × CO treatments (Figure 5G and H). Additionally, 1 × CO and 10 × CO but not 5 × CO remarkably suppressed the increase of p-I-κB in IVDD mice (Figure 5E and F).
5 × CO as an Optimal Level for Delaying IVDD Progression
To facilitate a more intuitive and comprehensive comparison of the therapeutic efficacy of 3 LWDHD formulations, we devised a scoring standard to assess their combined efficacy within IVD tissues (refer to Table S1). After computing the total score for each LWDHD formulation, and the results revealed that 5 × CO obtained the highest score (Table 2), implying that LWDHD containing this particular dosage may represent the most effective option for delaying the progression of IVDD.
![]() |
Table 2 Combined Score of Modified Formulations of LWDHD in Delaying IVDD |
Discussion
IVDD is a clinical common problem characterized by impaired structural integrity with reduced NP cell viability, diminished ECM matrix content, inflammation, and nerve ingrowth,22 lacking effective disease-modifying therapies. LWDHD has historically been a prominent prescription in TCM, historically utilized for various kidney yin deficiency-induced conditions, including LBP. Our earlier studies have highlighted the potential of LWDHD, including its component CO-derived compound morroniside, for the treatment of IVDD disorder by protecting NP cells from injury,8 and suggesting loganin and morroniside, two CO’s active components, in mitigating knee joint degeneration by targeting chondrocyte pyroptosis.9,10 These findings collectively suggest anti-inflammatory and anti-pyroptosis properties of CO in treating degenerative osteoarthropathies. However, uncertainty remains regarding the original LWDHD formulation’s optimal CO dosage and its potential mechanism for effectively treating IVDD. In the present study, we compared the therapeutic efficacy of 2 modified formulations of LWDHD containing varying doses of CO (5- or 10-time original dosage) (referred to as 5 × CO and 10 × CO, respectively) against the original LWDHD formulation using LSI surgery-induced IVDD mice. Our present results demonstrated that the administration of modified LWDHD formulations, particularly with 5 × CO, exhibited notable improvements in lumbar function, structural integrity, ECM composition, NP cell viability, and sensory nerve ingrowth in IVDs of IVDD mice. Importantly, 5 × CO formulation particularly suppressed NP pyroptosis by activating NRF2/KEAP1 pathway, offering promising evidence for its potential as an effective therapeutic approach to mitigate IVDD progression (Figure 6). This presents a robust rationale for considering LWDHD, specifically with the 5 × CO formulation, as a potential intervention strategy for IVDD.
Morroniside, one of the main iridoid glycosides in CO, has been shown in previous research to significantly improve the anti-oxidation and anti-apoptosis capabilities of mice liver when administered at doses of 20 mg/kg and 100 mg/kg body weight per day for 8 weeks, with the 100 mg/kg dose outperforming the 20 mg/kg dose.23 Based on these findings, we hypothesized that a similar dose-dependent effect might be observed in IVD tissue if the dosage of CO is increased. Therefore, we decided to test a dosage that is 5 times higher than the original prescription to evaluate this potential effect. Additionally, to further investigate the dose-dependency of CO’s therapeutic effects, we included a group with a dosage that is 10 times the original prescription. This experimental design allows us to comprehensively assess the efficacy of CO at varying dosages in the modified LWDHD formulations.
Pyroptosis, a form of programmed cell death that is facilitated by CASPASE1, stimulates cells to release inflammatory factors, including IL-1β, thereby initiating pro-inflammatory reactions in adjacent cells.24 Differing from other types of cell death, such as apoptosis, necrosis, and autophagy, its distinctive features encompass pore formation on the plasma membrane, cellular swelling and rupture, followed by the release of inflammatory cytokines (eg, IL-1β and IL-18) and intracellular materials.24 Previous studies have revealed that the heightened levels of IL-1β resulting from pyroptosis have been linked to impediments in ECM synthesis, which in turn facilitates the degradation of ECM and induces apoptosis in IVD cells.16 However, it remains uncertain whether the inhibition of pyroptosis can be effective against IVDD. Our findings discovered that the utilization of the original formulation of LWDHD has the potential to mitigate pyroptosis, and increasing the dosage of CO by a factor of 5 could further improve the anti-pyroptosis effect by further lowering the level of IL-1β. Notably, the contradiction arises from the lack of significant differences in the levels of CASPASE1 and GSDMD between 1× CO-treated mice and 5 × CO-treated mice, suggesting that the superior improvement of IL-1β by 5 × CO may be attributed to the targeting of other pyroptosis-related molecules, such as NOD-like receptor thermal protein domain associated protein 3 (NLRP3). Subsequently, we aim to conduct further experiments to confirm this.
Pyroptosis can be induced by various stimuli, including oxidative stress.25 The nuclear factor NRF2/KEAP1 pathway plays a crucial role in regulating the body’s antioxidant status. The latest findings from our work and others have revealed a strong association between NRF2/KEAP1 pathway dysfunction and the development of various spinal-related diseases including IVDD and osteoporosis, and thyroid toxicity.17,18,26,27 Consistent with these findings, our results demonstrated that LWDHD containing varying CO significantly upregulated the decreased NRF2 (S40) but lowered the increased KEAP1 expression in NP tissues, except for a higher upregulation in KEAP1 in 10 × CO-treated mice. These results suggest that moderately increasing the CO dose in LWDHD can achieve better anti-oxidant and anti-pyroptosis effects on NP cells.
While our findings are groundbreaking, it is important to acknowledge several limitations. First, the relatively short study duration of 8 weeks did not allow for the assessment of long-term treatment effects and potential side effects. To address these issues, we intend to further investigate the efficacy and potential side effects of IVDD disorder after 12 and 24 weeks of treatment with LWDHD formulations. Moreover, in our previous study, a total of 71 active ingredients of LWDHD were initially selected based on specific screening criteria, including oral bioavailability (OB) ≥30% and drug-likeness (DL) ≥0.18, after retrieving the TCM Systems Pharmacology Database and Analysis Platform (http://tcmspw.com/tcmsp.php).27 However, it remains uncertain whether the overall effectiveness of TCM formulation is inferior or superior to the combination of active ingredients in this formulation. Consequently, in future experiments, we intend to conduct further comparative investigations on the efficacy of formulation with the combination of active ingredients in this formulation. Furthermore, the detailed molecular mechanism explaining this therapeutic effect of modified LWDHD formulation is not fully elaborated. We will further confirm the specific mechanism of NRF2-mediated NP cell apoptosis in LWDHD intervention for the treatment of IVDD using transgenic animal and in vitro NP cell models. The implementation of all these aspects will significantly enhance our understanding of the therapeutic efficiency of LWDHD for IVDD disorder.
Conclusions
In summary, our findings provide valuable considerations for optimizing the formulation of LWDHD in the management of IVDD. Comprehensive therapeutic efficacy score analysis suggests that the 5 × CO is a superior modified formulation for treating IVDD, demonstrating better improvement in NP cell pyroptosis, reduction in sensory innervation, and enhancement of overall lumbar spine function.
Acknowledgments
This research was funded by National Natural Science Foundation of China (82174140, 82174401, 82104164), Natural Science Foundation of Zhejiang Province (LY22H270003 and LQ23H270003), the Joint Funds of the Zhejiang Provincial Natural Science Foundation of China under Grant No. LBY22H270008 and LBY21H060002, Traditional Chinese Medical Administration of Zhejiang Province (2023ZR019, 2022ZX005, 2022ZB119), Medical Science and Technology Project of Zhejiang Province (2023RC194, 2024KY1216, 2023KY235, and 2021KY222), Research Project of Zhejiang Chinese Medical University (2023JKZKTS40, 2021JKZDZC02), Research Project of Zhejiang Chinese Medical University Affiliated Hospital (2022FSYYZZ05, 2023FSYYZZ02, 2023FSYYZY40, and 2022FSYYZQ02).
Disclosure
The authors report no conflicts of interest in this work.
References
1. Wu A, March L, Zheng X, et al. Global low back pain prevalence and years lived with disability from 1990 to 2017: estimates from the Global Burden of Disease Study 2017. Ann Transl Med. 2020;8(6):299. doi:10.21037/atm.2020.02.175
2. Peng BG. Pathophysiology, diagnosis, and treatment of discogenic low back pain. World J Orthop. 2013;4(2):42–52. doi:10.5312/wjo.v4.i2.42
3. Peng B, Du L, Zhang T, Chen J, Xu B. Research progress in decellularized extracellular matrix hydrogels for intervertebral disc degeneration. Biomater Sci. 2023. doi:10.1039/d2bm01862d
4. Mohd Isa IL, Teoh SL, Mohd Nor NH, Mokhtar SA. Discogenic low back pain: anatomy, pathophysiology and treatments of intervertebral disc degeneration. Int J Mol Sci. 2022;24(1). doi:10.3390/ijms24010208
5. Ozaki Y, Reinhard JF, Nichol CA. Cofactor activity of dihydroflavin mononucleotide and tetrahydrobiopterin for murine epididymal indoleamine 2,3-dioxygenase. Biochem Biophys Res Commun. 1986;137(3):1106–1111. doi:10.1016/0006-291x(86)90339-6
6. Ge JR, Xie LH, Chen J, et al. Liuwei Dihuang Pill () treats postmenopausal osteoporosis with Shen (Kidney) Yin Deficiency via janus kinase/signal transducer and activator of transcription signal pathway by up-regulating cardiotrophin-like cytokine factor 1 expression. Chin J Integr Med. 2018;24(6):415–422. doi:10.1007/s11655-016-2744-2
7. Zhang H, Yao S, Zhang Z, et al. Network pharmacology and experimental validation to reveal the pharmacological mechanisms of liuwei dihuang decoction against intervertebral disc degeneration. Drug Des Devel Ther. 2021;15:4911–4924. doi:10.2147/DDDT.S338439
8. Zhou C, Yao S, Fu F, et al. Morroniside attenuates nucleus pulposus cell senescence to alleviate intervertebral disc degeneration via inhibiting ROS-Hippo-p53 pathway. Front Pharmacol. 2022;13:942435. doi:10.3389/fphar.2022.942435
9. Hu J, Zhou J, Wu J, et al. Loganin ameliorates cartilage degeneration and osteoarthritis development in an osteoarthritis mouse model through inhibition of NF-κB activity and pyroptosis in chondrocytes. J Ethnopharmacol. 2020;247:112261. doi:10.1016/j.jep.2019.112261
10. Yu H, Yao S, Zhou C, et al. Morroniside attenuates apoptosis and pyroptosis of chondrocytes and ameliorates osteoarthritic development by inhibiting NF-κB signaling. J Ethnopharmacol. 2021;266:113447. doi:10.1016/j.jep.2020.113447
11. Chen Y, Wu Y, Gan X, et al. Iridoid glycoside from Cornus officinalis ameliorated diabetes mellitus-induced testicular damage in male rats: involvement of suppression of the AGEs/RAGE/p38 MAPK signaling pathway. J Ethnopharmacol. 2016;194:850–860. doi:10.1016/j.jep.2016.10.079
12. Dong Y, Feng ZL, Chen HB, Wang FS, Lu JH. Corni Fructus: a review of chemical constituents and pharmacological activities. Chin Med. 2018;13:34. doi:10.1186/s13020-018-0191-z
13. Ge Y, Chen Y, Guo C, et al. Pyroptosis and intervertebral disc degeneration: mechanistic insights and therapeutic implications. J Inflamm Res. 2022;15:5857–5871. doi:10.2147/JIR.S382069
14. Li H, Wang X, Pan H, et al. The mechanisms and functions of IL-1β in intervertebral disc degeneration. Exp Gerontol. 2023;177:112181. doi:10.1016/j.exger.2023.112181
15. Lyu FJ, Cui H, Pan H, et al. Painful intervertebral disc degeneration and inflammation: from laboratory evidence to clinical interventions. Bone Res. 2021;9(1):7. doi:10.1038/s41413-020-00125-x
16. Fu F, Bao R, Yao S, et al. Aberrant spinal mechanical loading stress triggers intervertebral disc degeneration by inducing pyroptosis and nerve ingrowth. Sci Rep. 2021;11(1):772. doi:10.1038/s41598-020-80756-6
17. Chen Y, Cao X, Pan B, et al. Verapamil attenuates intervertebral disc degeneration by suppressing ROS overproduction and pyroptosis via targeting the Nrf2/TXNIP/NLRP3 axis in four-week puncture-induced rat models both in vivo and in vitro. Int Immunopharmacol. 2023;123:110789. doi:10.1016/j.intimp.2023.110789
18. Ma H, Xie C, Chen Z, et al. MFG-E8 alleviates intervertebral disc degeneration by suppressing pyroptosis and extracellular matrix degradation in nucleus pulposus cells via Nrf2/TXNIP/NLRP3 axis. Cell Death Discov. 2022;8(1):209. doi:10.1038/s41420-022-01002-8
19. Kilkenny C, Browne WJ, Cuthill IC, Emerson M, Altman DG. Improving bioscience research reporting: the ARRIVE guidelines for reporting animal research. PLoS Biol. 2010;8(6):e1000412. doi:10.1371/journal.pbio.1000412
20. Norcross JP, Lester GE, Weinhold P, Dahners LE. An in vivo model of degenerative disc disease. J Orthop Res. 2003;21(1):183–188. doi:10.1016/S0736-0266(02)00098-0
21. Ura K, Yamada K, Tsujimoto T, Ukeba D, Iwasaki N, Sudo H. Ultra-purified alginate gel implantation decreases inflammatory cytokine levels, prevents intervertebral disc degeneration, and reduces acute pain after discectomy. Sci Rep. 2021;11(1):638. doi:10.1038/s41598-020-79958-9
22. Vergroesen PP, Kingma I, Emanuel KS, et al. Mechanics and biology in intervertebral disc degeneration: a vicious circle. Osteoarthritis Cartilage. 2015;23(7):1057–1070. doi:10.1016/j.joca.2015.03.028
23. Park CH, Noh JS, Kim JH, et al. Evaluation of morroniside, iridoid glycoside from Corni Fructus, on diabetes-induced alterations such as oxidative stress, inflammation, and apoptosis in the liver of type 2 diabetic db/db mice. Biol Pharm Bull. 2011;34(10):1559–1565. doi:10.1248/bpb.34.1559
24. Li Y, Jiang Q. Uncoupled pyroptosis and IL-1β secretion downstream of inflammasome signaling. Front Immunol. 2023;14:1128358. doi:10.3389/fimmu.2023.1128358
25. Shao F. Gasdermins: making pores for pyroptosis. Nat Rev Immunol. 2021;21(10):620–621. doi:10.1038/s41577-021-00602-2
26. Chen Y, Zhou C, Bian Y, et al. Cadmium exposure promotes thyroid pyroptosis and endocrine dysfunction by inhibiting Nrf2/Keap1 signaling. Ecotoxicol Environ Saf. 2023;249:114376. doi:10.1016/j.ecoenv.2022.114376
27. Fu F, Luo H, Du Y, et al. AR/PCC herb pair inhibits osteoblast pyroptosis to alleviate diabetes-related osteoporosis by activating Nrf2/Keap1 pathway. J Cell Mol Med. 2023;27(22):3601–3613. doi:10.1111/jcmm.17928