Fitness
Efficacy and Safety Factors Related to Plasma Concentration-Optimized | IDR
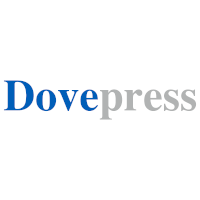
Introduction
Carbapenem-resistant Gram-negative bacterial (CR-GNB) infections are an urgent global public health threat with a high mortality rate1 and are mainly caused by carbapenem-resistant Enterobacteriaceae (CRE), Pseudomonas aeruginosa (CRPA), Acinetobacter baumannii (CRAB), and Klebsiella pneumoniae (CRKP).2 The emergence of these bacteria is a great challenge to clinical anti-infective therapy, and rational selection of antimicrobial drugs has become an important method to prevent and treat CR-GNB infections.
Polymyxin B (PMB) is one of the treatment options used for CR-GNB infections. Intravenous PMB is used to treat serious infections caused by a variety of pathogens, such as bacteremia, postoperative wound infections, and abdominal infections.3–6 Previous studies have shown that PMB might be ineffective against pneumonia because of its large molecular structure and difficulty penetrating the pleura.7 Recent findings suggested that aerosol inhalation delivery may have a positive effect on pneumonia when intravenous PMB fails.8,9
Administration of intravenous polymyxin B may lead to significant adverse effects in patients, such as nephrotoxicity, neurotoxicity, and skin darkening. Acute kidney injury (AKI) is the most concerning PMB toxicity issue that tends to limit its clinical application. Pharmacokinetic/toxicodynamic (PK/PD) data for PMB are scarce, and direct quantitative data establishing exposure-toxicity relationships are lacking. International consensus guidelines9 recommend a daily dose of 1.25–1.50 mg/kg (q12h) for PMB, with a recommended loading dose of 2.0–2.5 mg/kg; however, high doses can cause nephrotoxicity. In a study by Elias,10 doses of PMB greater than 200 mg/day increased the risk of nephrotoxicity while decreasing in-hospital mortality. From a safety perspective, clinicians are usually reluctant to select higher doses of PMB to avoid aggravating renal impairment; however, whether reducing the dose of PMB results in reduced in vivo antimicrobial activity at therapeutic blood levels is unknown. Because of this, there is an urgent need to understand the effective concentration range and renal toxicity concentration of PMB in patients with CR-GNB infections with high mortality rates, especially those with abnormal renal function.
Area under the concentration-time curve (AUC) thresholds of 50–100 mg h /L have been reported to reduce nephrotoxicity while ensuring clinical efficacy.11 In a prospective study, the AUC/Minimal Inhibitory Concentration (MIC) threshold for the efficacy of PMB in patients with pneumonia was 66.9.12 Despite the recognized value of PMB in treating CR-GNB infections, many questions regarding its optimal use, efficacy, and safety remain unanswered, including which factors are associated with the efficacy and safety of PMB. As PMB is increasingly being used to treat CR-GNB-induced infections, more studies are urgently needed to guide rational clinical use. In this study, we adjusted the PMB regimens by therapeutic drug monitoring (TDM) and estimated AUC, exploring the clinical efficacy and safety monitoring indices of PMB combination therapy. The results can help to develop a more rational clinical dosing regimen to improve antimicrobial efficacy and reduce nephrotoxicity.
Materials and Methods
Ethical Approval of the Study Protocol
The study was approved by the Ethics Committees of Xinhua Hospital, School of Medicine, Shanghai Jiao Tong University (No. XHEC-D-2024-014). It was carried out by the ethical standards laid down in the 1964 Declaration of Helsinki and its later amendments. Due to the retrospective nature of the study, the study had no impact on the existed treatment. No biospecimens were collected from patients in this study. The confidentiality of patients’ privacy and identity information was guaranteed, and there was no commercial interest involved, so that this research met the conditions for exemption from informed consent.
Patient Enrollment and Data Collection
An observational study on hospitalized patients treated with intravenous PMB was conducted at the Xinhua Hospital School of Medicine (Shanghai Jiao Tong University, Shanghai, China) from January 2021 to September 2023. Inclusion criteria were that patients (i) who had a PMB medication history (Shanghai First Biochemical Pharmaceutical Co., Ltd., Shanghai, China), (ii) were infected with CR-GNB based on bacterial culture results, and (iii) had complete medical data and basic information. The exclusion criteria were (i) PMB was administered for less than 72 hours (ii) the Cmin and Cmax of PMB were not monitored (iii) Patients who had received chemotherapy drugs for anti-tumor treatment before or during PMB treatment. Data were extracted from electronic medical records including demographics, comorbidities, causative organisms, medications, physiologic parameters, and laboratory values for the day of initiation of PMB as well as during treatment.
Definitions
On the day of drug administration, Sequential Organ Failure Assessment (SOFA) and biochemical markers of infection, such as white blood cell count (WBC), C-reactive protein (CRP), and procalcitonin (PCT), along with liver and kidney function indicators, were established as baseline measures. Subsequently, these markers were assessed twice weekly for evaluating therapeutic efficacy. Infections were diagnosed by two physicians based on clinical symptoms, microbiological cultures, and radiological findings. The identification of pathogenic bacteria was carefully differentiated from colonization and contamination in the absence of infection. Surgery was defined as any invasive procedures documented in the surgical record in the electronic medical records. The use of vasoactive drugs was characterized by the administration of agents such as dobutamine, norepinephrine, and dopamine, which are commonly employed in anti-shock therapy. The susceptibility of the causative Gram-negative bacilli (GNB) to carbapenems was determined following the guidelines of the European Committee on Antimicrobial Susceptibility Testing (EUCAST). The EUCAST breakpoints for PMB against the mentioned bacterial strains were defined as 2 mg/L or lower for susceptibility and greater than 2 mg/L for resistance. Patients were monitored for two weeks following PMB discontinuation.
Polymyxin B Administration and Therapeutic Drug Monitoring
Therapeutic management was determined by the physician, including dosage, infusion schedule, timing of administration, adjustment of therapy, and concomitant use of antibiotics. Peak and trough concentrations of PMB were monitored after 4–5 doses. Blood samples were collected twice, before (C0.5 hours) and 2 hours after (C2 hours) the start of the infusion. Plasma concentrations of PMB were determined using a previously published validated ultra-high performance liquid chromatography-tandem mass spectrometry method.13 The two concentrations (peak and trough) and a simple analytical equation were used to estimate AUC values.14 AUC of 50–100 mg· h/L was recommended for the treatment of CR-GNB.9 Clinical pharmacists provided PMB adjustment regimen reports based on estimated AUC values. Clinicians adjusted the PMB regimens based on the TDM report. Specific methods included increasing the single dose of PMB if the AUC did not reach the target value, and decreasing the dose of PMB or reducing the dosing frequency if the AUC target value was exceeded.
Outcome Measurement
The primary endpoints were clinical efficiency and incidence of AKI associated with PMB. The efficacy criteria were treatment success (including normalization or improvement of clinical symptoms, signs, laboratory test results, and imaging tests after treatment) or failure (with no significant improvement of the above, deterioration of the condition, or a switch to other treatment options). Creatinine clearance (Clcr) in adults was calculated using the Cockcroft Gault formula; the pediatric estimation glomerular filtration rate (eGFR) was used with a creatinine-based formula (creatinine – blood urea nitrogen).15 Clcr ≥ 90 mL/min indicated normal renal function. AKI was defined as an increase in serum creatinine of 0.3 mg/L (26.5 μmol/L) and 50% from baseline on two consecutive occasions during the treatment period. The two creatinine values in this study were measured on the day of drug administration and 7 days after PMB administration.
Secondary endpoints were 30-day all-cause mortality, bacterial clearance of PMB, and skin pigmentation. The 30-day all-cause mortality was recorded from the start of PMB treatment. Pathogenic microbiological cultures were conducted twice weekly, with bacterial clearance evaluations at 7 and 14 days post-treatment. Bacterial clearance was evaluated as clearance with two consecutive bacterial cultures showing negative results or two consecutive bacterial cultures showing growth of other bacteria and disappearance of the original pathogen; non-clearance was defined as bacterial cultures still showing the original pathogen.
Statistical Analyses
Statistical analyses were performed using SPSS v21.0. Data were expressed as median and interquartile range (IQR) for continuous variables and as frequency / percentage (%) for categorical variables. Logistic regression was used to analyze the relationships between variables with clinical efficacy, bacterial clearance rate, and adverse drug reaction (ADR), and reported as estimated as odds ratios (ORs). In addition, 30-day mortality factors were analyzed by Cox regression and estimated by hazard ratios (HRs). In the univariate analysis, variables with a p-value less than 0.1 were selected for inclusion in the multivariate regression model. The backward elimination method was then applied in SPSS to identify the significant factors associated with the outcome. The sensitivity and specificity of Cmin and AUC for the occurrence of AKI were determined by ROC curve analysis and AKI occurrence as the outcome, finding high sensitivity and specificity index became the best entry point. Chi-square test analyzed categorical variables. All results were considered significant at P
Results
Patient Demographics and Clinical Outcomes
A cohort of 168 patients was administered PMB and underwent TDM during the study period. After excluding 38 patients who met the exclusion criteria, 130 patients were included in the final analysis. The median age was 67 years (IQR: 51–78 years). The age distribution included 18.5% of patients 80 years, with a male predominance of 67.7%. The most prevalent site of infection was the lung, accounting for 64.6% of cases (84/130), followed by bloodstream (40%, 52/130) and abdominal infections (15.4%, 20/130). Notably, 20.8% of the patients (27/130) presented with multi-site infections. Adverse drug reactions during medication included 14.6% of AKI cases (19/130) and 50.8% of skin pigmentation cases (66/130). Detailed information regarding patient demographics and clinical outcomes is presented in Table 1.
Table 1 Patients Demographics and Clinical Outcomes |
Polymyxin B Exposure and Drug Concentration Monitoring results
For adults, the loading dose of PMB was 1.7 mg/kg (IQR: 1.5–2.0 mg/kg), while the maintenance dose was 0.9 mg/kg (IQR: 0.8–1.0 mg/kg); in children, these doses were 1.6 mg/kg (IQR: 1.5–2.0 mg/kg) and 1.1 mg/kg (IQR: 1.0–1.3 mg/kg), respectively. PMB was administered by aerosol inhalation in 33 cases, and intrathecal injection was used in two.
Out of the 130 patients in the study, 107 were adults and 23 were children. Among them, 83 patients underwent blood sample testing once, with 2 samples taken each time to measure trough and peak concentrations. Additionally, 47 patients had their blood samples tested more than 2 times, resulting in a total of 382 blood samples being tested 191 times. The Cmin observed was 2.4 µg/mL (IQR: 1.8–3.5 µg/mL) in adults and 0.8 µg/mL (IQR: 0.6–1.3 µg/mL) in children. The Cmax was 5.4 µg/mL (IQR: 4.3–7.0 µg/mL) for adults and 4.4 µg/mL (IQR: 3.4–6.3 µg/mL) for children. The AUC values were 91.8 mg h/L (IQR: 70.8–119.6 mg h/L) in adults and 55.8 mg h/L (IQR: 44.5–77.9 mg h/L in children. Detailed data of 191 times of PMB drug concentration monitoring are presented in Table S1. Out of the total 43 patients, the PMB regimens were modified based on AUC estimation. The initial concentrations of PMB in the patients were used in several studies to analyze factors affecting the clinical efficacy of PMB, bacterial clearance rate, 30-day all-cause mortality, AKI, skin pigmentation, and ROC curve analysis.
Analysis of Factors Affecting Clinical Efficacy of Polymyxin B
The overall clinical response rate to PMB was 65.4% (85/130). Univariate logistic regression analysis identified several factors influencing the clinical efficacy of PMB, including bloodstream infection (OR: 4.10; 95% CI: 1.759–9.535; P = 0.001), lung infection (OR: 0.22; 95% CI: 0.087–0.541; P = 0.001), CRRT (OR: 0.27; 95% CI: 0.090–0.789; P = 0.017), CRP (OR: 0.32; 95% CI: 0.140–0.725; P = 0.006), and Coronavirus Disease 2019 (COVID-19) (OR: 0.30; 95% CI: 0.101–0.918; P = 0.035). Multivariate logistic regression analysis revealed that lung infection, CRRT, and CRP were independent factors significantly associated with the clinical efficacy of PMB (Table 2). The study included 84 patients with lung infection, with 16 cases in the effective group and 17 cases in the ineffective group receiving PMB atomization treatment. The chi-square test yielded a p-value of 0.352, suggesting that there was no statistically significant difference between the two groups.
![]() |
Table 2 Univariate and Multivariate Analysis of Clinical Efficacy of Polymyxin B |
Analysis of Factors Influencing 30-Day All-Cause Mortality
The 30-day all-cause mortality rate was found to be 30% (39/130). Univariate Cox regression analysis showed that age, bloodstream infection, lung infection, CRP, use of vasopressors, CRRT, and combined COVID-19 were the main influencing factors. Bloodstream infection (HR: 0.30; 95% CI: 0.122–0.713; P = 0.007), use of vasopressors (HR: 2.12; 95% CI: 1.086–4.138; P = 0.028), CRP (HR: 3.45; 95% CI: 1.752–6.803; P = 0.000), and COVID-19 (HR: 2.48; 95% CI: 1.151–5.354; P = 0.020) were identified as independent risk factors for 30-day all-cause mortality (Table 3). Kaplan–Meier survival estimates for 30-day all-cause mortality are illustrated in Figure 1.
![]() |
Table 3 Univariate and Multivariate Analysis of 30-Day All-Cause Mortality |
Analysis of Factors Affecting Bacterial Clearance
The total bacterial clearance rate was 53.9% (70/130), occurring within 7 days in 26.9% of cases (35/130). Univariate logistic analysis identified four statistically significant factors: mechanical ventilation, bloodstream infection, lung infection, and surgery. Multivariate logistic regression analysis revealed that bloodstream infection (OR: 5.58; 95% CI: 2.45–12.71; P Table S2.
Analysis of Factors Influencing Acute Kidney Injury and Skin Pigmentation During Polymyxin B Treatment
Acute kidney injury was observed in 14.6% of patients (16/130) during PMB treatment. Univariate logistic analysis identified age, Cmin, AUC, combined carbapenem use, and combined piperacillin-tazobactam as factors with significant differences between those who developed AKI and those who did not. Multivariate analysis revealed that age > 73 years (OR: 3.63; 95% CI: 1.035–12.727; P = 0.044), Cmin greater than 2.3 µg/mL (OR: 7.37; 95% CI: 1.571–34.580; P = 0.011), combined vancomycin (OR: 9.47; 95% CI: 1.732–51.731; P = 0.009), and combined piperacillin-tazobactam (OR: 21.87; 95% CI: 3.139–152.324; P = 0.002) were independent risk factors for AKI (Table 4).
![]() |
Table 4 Analysis of Factors Influencing Acute Kidney Injury During Polymyxin B Treatment |
In the analysis of skin pigmentation, PMB treatment duration and age were identified as independently associated risk factors, with odds ratios of 44.788 (95% CI: 13.724–146.162; P Table S3).
Determining the Polymyxin B Cut-off Values of Cmin and AUC24 for Predicting Acute Kidney Injury During Polymyxin B Treatment
In this study, we attempted to use ROC curve analysis to establish the cut-off values for the Cmin and AUC24 of PMB that predicted the occurrence of AKI. The identified cut-off values were Cmin at 2.3 µg/mL (sensitivity: 78.9%; specificity: 61.3%) and AUC24 at 82.0 mg· h/L (sensitivity: 84.2%; specificity: 52.3%). Cmin demonstrated a superior predictive value compared with AUC24, as evidenced by a larger area under the ROC curve (0.73 with 95% CI: 0.635–0.800 and 0.69 with 95% CI: 0.592–0.791, respectively) (Figure 2).
![]() |
Figure 2 Receiver operating characteristic curve of the Cmin and AUC24 of PMB for AKI. Abbreviations: Cmin, Trough concentration; AUC24, Area Under the concentration-time Curve across 24 h. |
Discussion
In this study, we analyzed the clinical efficacy of PMB-based combination regimens for the treatment of CR-GNB infections. Factors including lung infection and CRRT treatment were identified as unfavorable, while CRP levels 73 years, Cmin > 2.3 µg/mL, and combination therapy with either piperacillin-tazobactam or vancomycin were independent risk factors for AKI. Cmin ≥ 2.3 µg/mL and AUC ≥ 82.0 mg h/L were the cutoff values for predicting PMB-associated AKI.
The clinical efficacy of PMB was 65.4%, and increasing PMB concentration did not affect efficacy. There was no correlation between clinical efficacy and PMB dose in this study, but many previous studies have shown that clinical efficacy was associated with high doses of PMB.10,16,17 Here, the median daily dose of PMB was 1.0 mg/kg (q12h), which was lower than the guideline-recommended dose. Cmin /MIC, Cmax /MIC, and AUC/MIC were not associated with the clinical efficacy of PMB; however, the effective range of trough concentration and Cmin /MIC of PMB in combination with other antibiotics still need to be studied and observed in large samples. In this study, PMB-based combination regimens were utilized for the treatment of CR-GNB infections, with carbapenems being the primary regimen. Other agents such as Cefoperazone Sodium Sulbactam, Tigecycline, and Fosfomycin were also included. There was no statistically significant difference in clinical efficacy between the various combined regimens. Several studies have demonstrated that for CR-GNB infections, PMB combination regimens containing carbapenems and other agents can lead to improved clinical efficacy and microbial response.18–21This study further confirmed that PMB-based combination regimens are effective in treating CR-GNB infections, resulting in better clinical outcomes and microbial responses.
The clinical response rate of PMB for pneumonia was lower than for other sites of infection, and the 30-day all-cause mortality rate was higher for COVID-19 infection. It has been suggested that PMB combination therapy is effective for treating CRO pneumonia.22–24 Here, lung infections accounted for 63.1% of the cases, and there were combinations of infections at other sites in some cases, which might be partly responsible for the poor outcome of lung infections. Pharmacodynamic studies have shown that even with the highest tolerated dose of polymyxin, lung concentrations of the drug may be lower than optimal for the infecting strains unless the MICs of these pathogens were well below the breakpoint;25,26 inhaled polymyxins were recommended to overcome these drawbacks.9,27 In this study, only 33 patients with lung infection were treated with PMB aerosol inhalation, showing no significant difference in efficacy compared to those who did not undergo aerosol treatment. Variations in aerosol inhalation regimens and devices may impact the effectiveness of PMB, possibly contributing to the observed low efficacy in treating lung infections. Severe COVID-19 infection may increase the level of immunosuppression, possibly leading to more deaths in the later stages of hospitalization.28 The mortality rate in patients with COVID-19-MDR-GNB has been reported to be 68.6%.29 Additionally, 15 cases of COVID-19 infection in this study were complicated by CR-GNB, which could explain the high treatment failure rate in lung infections. Further research and analysis are needed to determine if high-dose intravenous PMB and aerosolized PMB are necessary to achieve adequate pulmonary drug concentrations in COVID-19 patients.
Guidelines recommend that patients undergoing continuous renal replacement therapy (CRRT) should not adjust the dose of PMB.9 Patients undergoing continuous veno-venous hemodiafiltration (CVVHDF) exhibit higher total clearance and a shorter terminal half-life of PMB.30 Some clinical studies suggest that higher doses of PMB could potentially improve efficacy and reduce mortality in patients undergoing renal replacement therapy.31,32 Our study involving 16 patients treated with CRRT and receiving the usual PMB dosage showed low clinical effectiveness. The pharmacokinetic changes in CRRT patients are intricate, with various factors influencing anti-infective efficacy. Further prospective studies focusing on different renal replacement therapy modalities will offer insights into safe and effective PMB dosing regimens for treatment. PMB demonstrates rapid, concentration-dependent bacterial killing in vitro against a variety of Gram-negative organisms.33 Several reports indicated that PMB holds promise in the clinical treatment of bloodstream infections.34–36 The study confirmed that blood cultures turned negative within 3 days of starting therapy in all patients assessed for microbiological efficacy.37 Moreover, there was evidence suggesting that early administration of PMB may lower the mortality rate in cases of CRKP bloodstream infections.38 The study also observed high bacterial clearance at the site of bloodstream infection across the range of drug concentrations tested, highlighting the clinical benefits of PMB in treating CR-GNB bloodstream infections.
The incidence of AKI was 14.6%, which was lower than the previously reported 20%-60%.39–41 Although the clearance of PMB did not depend on the glomerular filtration rate, 90–95% of filtered PMB was reabsorbed by tubular cells in critically ill patients.42,43 Because of reabsorption and intracellular accumulation, exposure of renal tubular cells to high concentrations of PMB can cause direct damage.42,44 A meta-analysis showed that being elderly, high daily dosage, having an underlying disease such as diabetes, and concomitant use of nephrotoxic drugs were independent predictors of polymyxin-induced nephrotoxicity.45 Our findings suggested that age > 73 years and the combined use of piperacillin-tazobactam or vancomycin were independent risk factors for AKI, consistent with the findings of the above-mentioned study. Regardless of initial renal function, routine adjustment of PMB dose by TDM avoided potential adverse outcomes, especially in elderly patients; this may also have contributed to the lower incidence of AKI. The AUC thresholds for PMB-associated AKI have been reported to be 99.4 mg h/L11 and 97.72 mg h/L.46 Maintaining PMB Cmin 47 Here, the risk of AKI was predicted to increase in combined PMB therapy when Cmin ≥ 2.3 µg /mL and AUC ≥ 82.0 mg h/L, much lower than the thresholds reported previously; this may be related to the higher proportion of older patients in our study population. Cmin was the better threshold for AKI prediction. Further clinical studies with large sample sizes are needed to concretely determine the efficacy of TDM for PMB in preventing AKI risk.
The prevalence of skin pigmentation was 50.8%, which is considerably higher than the previously reported 8 to 15%48,49 but it is reported that almost all patients treated with PMB developed hyperpigmentation on the head and neck.50 Most patients with hyperpigmentation had dark skin on the face and neck with skin changes appearing approximately a week after starting PMB treatments, but the degree of hyperpigmentation varied widely. This higher incidence may have been because we adopted a more stringent standard: darker skin on the face or neck than before treatment was considered to be skin hyperpigmentation. The exact pathogenesis of PMB-induced skin pigmentation is still debated and may be multifactorial. The cumulative effect of PMB in neonates and infants may affect skin darkening, most likely because of immature renal function.51 The cumulative effect of PMB in the body might also be a relevant factor. The present study confirms that younger patients have a greater likelihood of skin pigmentation, especially those
There are several limitations in the present study. No separate analysis was performed to characterize the PK/PD of PMB in patients who were children. The poor outcome of lung infections was influenced by many factors which were not systematically analyzed and summarized. Additionally, there was no further analysis of bacterial species and site of infection. These shortcomings will be further improved in follow-up studies.
Conclusion
Polymyxin B-based combination regimens are effective in the treatment of CR-GNB infections, especially in bloodstream infections where bacteria are easily cleared, but they have shown unsatisfactory efficacy in treating lung infections. Increasing the concentration of PMB did not affect efficacy within the range of doses and concentrations currently administered. Age > 73 years, Cmin ≥ 2.3 µg/mL, AUC ≥ 82.0 mg·h/L, combined therapy with vancomycin or with piperacillin-tazobactam led to a higher risk of AKI. Adjusting the PMB dose based on TDM is recommended to ensure adequate efficacy, though this needs further analysis of larger samples of cases.
Abbreviations
IQR, interquartile range; OR odds ratio; CI confidence interval; WBC, White Blood Cells; CRP, C-reactive protein; PCT, Procalcitonin; SOFA, Sequential Organ Failure Assessment; CRRT, continuous renal replacement therapy; ICU, intensive care unit; MIC, Minimal Inhibitory Concentration; COVID-19, Coronavirus Disease 2019; CRKP, Carbapenem-resistant Klebsiella Pneumoniae; CRAB, Carbapenem-resistant Acinetobacter baumannii; CRPA, Carbapenem-resistant Pseudomonas aeruginosa; Cmin, Trough concentration; Cmax, Peak concentration; AUC, Area Under the concentration-time Curve; TDM, Therapeutic drug monitoring; eGFR, estimated glomerular filtration rate. CR-GNB, Carbapenem-resistant Gram-negative bacterial.
Ethical Approval and Informed Consent
The study was conducted according to the guidelines of the Declaration of Helsinki, and approved by the Ethics Committees of Xinhua Hospital, School of Medicine, Shanghai Jiao Tong University (No. XHEC-D-2024-014). Patient consent was waived by the Ethics Committees (as confirmed by the ethics approval document we provided) due to retrospective data used in this study were fully desensitized.
Author Contributions
All authors made a significant contribution to the work reported, whether that is in the conception, study design, execution, acquisition of data, analysis and interpretation, or in all these areas; took part in drafting, revising or critically reviewing the article; gave final approval of the version to be published; have agreed on the journal to which the article has been submitted; and agree to be accountable for all aspects of the work.
Disclosure
The authors declare no conflicts of interest in this work.
References
1. Patel G, Bonomo RA. “Stormy waters ahead”: global emergence of carbapenemases. Front Microbiol. 2013;4:48. doi:10.3389/fmicb.2013.00048
2. Paul M, Daikos GL, Durante-Mangoni E, et al. Colistin alone versus colistin plus meropenem for treatment of severe infections caused by carbapenem-resistant Gram-negative bacteria: an open-label, randomised controlled trial. Lancet Infect Dis. 2018;18(4):391–400. doi:10.1016/s1473-3099(18)30099-9
3. Teng CB, Koh PT, Lye DC, et al. Continuous versus intermittent infusion of polymyxin B in the treatment of infections caused by multidrug-resistant Gram-negative bacteria. Int J Antimicrob Agents. 2008;31(1):80–82. doi:10.1016/j.ijantimicag.2007.08.004
4. Ouderkirk JP, Nord JA, Turett GS, et al. Polymyxin B nephrotoxicity and efficacy against nosocomial infections caused by multiresistant gram-negative bacteria. Antimicrob Agents Chemother. 2003;47(8):2659–2662. doi:10.1128/aac.47.8.2659-2662.2003
5. Parchuri S, Mohan S, Cunha BA. Extended spectrum beta-lactamase-producing Klebsiella pneumoniae chronic ambulatory peritoneal dialysis peritonitis treated successfully with polymyxin B. Heart Lung. 2005;34(5):360–363. doi:10.1016/j.hrtlng.2004.11.00
6. Sarria JC, Angulo-Pernett F, Kimbrough RC, et al. Use of intravenous polymyxin B during continuous venovenous hemodialysis. Eur J Clin Microbiol Infect Dis. 2004;23(4):340–341. doi:10.1007/s10096-004-1106-8
7. Pereira GH, Muller PR, Levin AS. Salvage treatment of pneumonia and initial treatment of tracheobronchitis caused by multidrug-resistant Gram-negative bacilli with inhaled polymyxin B. Diag Microbiol Infect Dis. 2007;58(2):235–240. doi:10.1016/j.diagmicrobio.2007.01.008
8. Falagas ME, Bliziotis IA, Tam VH. Intraventricular or intrathecal use of polymyxins in patients with Gram-negative meningitis: a systematic review of the available evidence. Int J Antimicrob Agents. 2007;29(1):9–25. doi:10.1016/j.ijantimicag.2006.08.024
9. Tsuji BT, Pogue JM, Zavascki AP, et al. International Consensus Guidelines for the Optimal Use of the Polymyxins: endorsed by the American College of Clinical Pharmacy (ACCP), European Society of Clinical Microbiology and Infectious Diseases (ESCMID), Infectious Diseases Society of America (IDSA), International Society for Anti-infective Pharmacology (ISAP), Society of Critical Care Medicine (SCCM), and Society of Infectious Diseases Pharmacists (SIDP). Pharmacotherapy. 2019;39(1):10–39. doi:10.1002/phar.2209
10. Elias LS, Konzen D, Krebs JM, et al. The impact of polymyxin B dosage on in-hospital mortality of patients treated with this antibiotic. J Antimicrob Chemother. 2010;65(10):2231–2237. doi:10.1093/jac/dkq285
11. Yang J, Liu S, Lu J, et al. An area under the concentration-time curve threshold as a predictor of efficacy and nephrotoxicity for individualizing polymyxin B dosing in patients with carbapenem-resistant gram-negative bacteria. Critical Care. 2022;26(1):320. doi:10.1186/s13054-022-04195-7
12. Thomas R, Velaphi S, Ellis S, et al. The use of polymyxins to treat carbapenem resistant infections in neonates and children. Exp Opin Pharmacother. 2019;20(4):415–422. doi:10.1080/14656566.2018.1559817
13. Wang P, Zhang Q, Qin Z, et al. A simple and robust liquid chromatography with tandem mass spectrometry analytical method for therapeutic drug monitoring of plasma and cerebrospinal fluid Polymyxin B1 and B2. Ther Drug Monit. 2020;42(5):716–723. doi:10.1097/ftd.0000000000000754
14. Pai MP, Neely M, Rodvold KA, et al. Innovative approaches to optimizing the delivery of vancomycin in individual patients. Adv Drug Delivery Rev. 2014;77:50–57. doi:10.1016/j.addr.2014.05.016
15. Pierce CB, Muñoz A, Ng DK, et al. Age- and sex-dependent clinical equations to estimate glomerular filtration rates in children and young adults with chronic kidney disease. Kidney Int. 2021;99(4):948–956. doi:10.1016/j.kint.2020.10.047
16. Ismail B, Shafei MN, Harun A, et al. Predictors of polymyxin B treatment failure in Gram-negative healthcare-associated infections among critically ill patients. J Microbiol Immunol Infect. 2018;51(6):763–769. doi:10.1016/j.jmii.2017.03.007
17. Vicari G, Bauer SR, Neuner EA, et al. Association between colistin dose and microbiologic outcomes in patients with multidrug-resistant gram-negative bacteremia. Clinl Infect Dis. 2013;56(3):398–404. doi:10.1093/cid/cis909
18. Xia GL, Jiang RL. Efficacy and safety of polymyxin B in carbapenem-resistant gram-negative organisms infections. BMC Infect Dis. 2021;21(1):1034. doi:10.1186/s12879-021-06719-y
19. Scudeller L, Righi E, Chiamenti M, et al. Systematic review and meta-analysis of in vitro efficacy of antibiotic combination therapy against carbapenem-resistant Gram-negative bacilli. Int J Antimicrob Agents. 2021;57(5):106344. doi:10.1016/j.ijantimicag.2021.106344
20. Samal S, Samir SB, Patra SK, et al. Polymyxin Monotherapy vs. Combination therapy for the treatment of multidrug-resistant infections: a systematic review and meta-analysis. Indian J Crit Care Med. 2021;25(2):199–206. doi:10.5005/jp-journals-10071-23720
21. Ardebili A, Izanloo A, Rastegar M. Polymyxin combination therapy for multidrug-resistant, extensively-drug resistant, and difficult-to-treat drug-resistant gram-negative infections: is it superior to polymyxin monotherapy? Exp Rev Anti-Infective Ther. 2023;21(4):387–429. doi:10.1080/14787210.2023.2184346
22. Tang T, Li Y, Xu P, et al. Optimization of polymyxin B regimens for the treatment of carbapenem-resistant organism nosocomial pneumonia: a real-world prospective study. Critical Care. 2023;27(1):164. doi:10.1186/s13054-023-04448-z
23. Qiao L, Zuo W, Yang Y, et al. Clinical outcomes and safety of intravenous polymyxin B-based treatment in critically ill patients with carbapenem-resistant Acinetobacter baumannii nosocomial pneumonia. Int J Antimicrob Agents. 2023;62(2):106880. doi:10.1016/j.ijantimicag.2023.106880
24. Lin H, Liu X, Sun P. Effects of aerosol inhalation combined with intravenous drip of polymyxin b on bacterial clearance, symptoms improvement, and serum infection indexes in patients with pneumonia induced by multidrug-resistant gram-negative bacteria. Emer Med Int. 2022;2022:5244538. doi:10.1155/2022/5244538
25. Landersdorfer CB, Wang J, Wirth V, et al. Pharmacokinetics/pharmacodynamics of systemically administered polymyxin B against Klebsiella pneumoniae in mouse thigh and lung infection models. J Antimicrob Chemother. 2018;73(2):462–468. doi:10.1093/jac/dkx409
26. Cheah SE, Wang J, Nguyen VT, et al. New pharmacokinetic/pharmacodynamic studies of systemically administered colistin against Pseudomonas aeruginosa and Acinetobacter baumannii in mouse thigh and lung infection models: smaller response in lung infection. J Antimicrob Chemother. 2015;70(12):3291–3297. doi:10.1093/jac/dkv267
27. Erb CT, Patel B, Orr JE, et al. Management of adults with hospital-acquired and ventilator-associated pneumonia. Ann Am Thoracic Soc. 2016;13(12):2258–2260. doi:10.1513/AnnalsATS.201608-641CME
28. Wu M, Zou ZY, Chen YH, et al. Severe COVID-19-associated sepsis is different from classical sepsis induced by pulmonary infection with carbapenem-resistant Klebsiella pneumonia (CrKP). Chin J Traumatol. 2022;25(1):17–24. doi:10.1016/j.cjtee.2021.11.001
29. de Souza GHA, de Oliveira AR, Dos Santos Barbosa M, et al. Multidrug-resistant gram-negative bacteria in patients with COVID-19: an epidemiological and clinical study. J Infect Pub Health. 2023;16(8):1184–1192. doi:10.1016/j.jiph.2023.05.017
30. Hanafin PO, Kwa A, Zavascki AP, et al. A population pharmacokinetic model of polymyxin B based on prospective clinical data to inform dosing in hospitalized patients. Clin Microbiol Infect. 2023;29(9):1174–1181. doi:10.1016/j.cmi.2023.05.018
31. Wang P, Xing H, Zhang F, et al. Population pharmacokinetics of polymyxin B in critically ill patients receiving continuous venovenous haemofiltration. Int J Antimicrob Agents. 2022;60(1):106599. doi:10.1016/j.ijantimicag.2022.106599
32. Rigatto MH, Falci DR, Lopes NT, et al. Clinical features and mortality of patients on renal replacement therapy receiving polymyxin B. Int J Antimicrob Agents. 2016;47(2):146–150. doi:10.1016/j.ijantimicag.2015.11.007
33. Poudyal A, Howden BP, Bell JM, et al. In vitro pharmacodynamics of colistin against multidrug-resistant Klebsiella pneumoniae. J Antimicrob Chemother. 2008;62(6):1311–1318. doi:10.1093/jac/dkn425
34. Kvitko CH, Rigatto MH, Moro AL, et al. Polymyxin B versus other antimicrobials for the treatment of pseudomonas aeruginosa bacteraemia. J Antimicrob Chemother. 2011;66(1):175–179. doi:10.1093/jac/dkq390
35. Medeiros GS, Rigatto MH, Falci DR, et al. Combination therapy with polymyxin B for carbapenemase-producing Klebsiella pneumoniae bloodstream infection. Int J Antimicrob Agents. 2019;53(2):152–157. doi:10.1016/j.ijantimicag.2018.10.010
36. Falcone M, Daikos GL, Tiseo G, et al. Efficacy of Ceftazidime-avibactam plus aztreonam in patients with bloodstream infections caused by Metallo-β-lactamase-producing enterobacterales. Clinl Infect Dis. 2021;72(11):1871–1878. doi:10.1093/cid/ciaa586
37. Yu Z, Liu X, Du X, et al. Pharmacokinetics/pharmacodynamics of polymyxin B in patients with bloodstream infection caused by carbapenem-resistant Klebsiella pneumoniae. Front Pharmacol. 2022;13:975066. doi:10.3389/fphar.2022.975066
38. Liang Q, Huang M, Xu Z. Early use of polymyxin B reduces the mortality of carbapenem-resistant Klebsiella pneumoniae bloodstream infection. Braz J Infect Dis. 2019;23(1):60–65. doi:10.1016/j.bjid.2018.12.004
39. Mattos KPH, Gouvêa IR, Quintanilha JCF, et al. Polymyxin B clinical outcomes: a prospective study of patients undergoing intravenous treatment. J Clin Pharm Therapeutics. 2019;44(3):415–419. doi:10.1111/jcpt.12801
40. Oliveira MS, Prado GV, Costa SF, et al. Polymyxin B and colistimethate are comparable as to efficacy and renal toxicity. Diag Microbiol Infect Dis. 2009;65(4):431–434. doi:10.1016/j.diagmicrobio.2009.07.018
41. Rigatto MH, Oliveira MS, Perdigão-Neto LV, et al. Multicenter prospective cohort study of renal failure in patients treated with colistin versus Polymyxin B. Antimicrob Agents Chemother. 2016;60(4):2443–2449. doi:10.1128/aac.02634-15
42. Sandri AM, Landersdorfer CB, Jacob J, et al. Population pharmacokinetics of intravenous polymyxin B in critically ill patients: implications for selection of dosage regimens. Clinl Infect Dis. 2013;57(4):524–531. doi:10.1093/cid/cit334
43. Zavascki AP, Nation RL. Nephrotoxicity of polymyxins: is there any difference between colistimethate and Polymyxin B? Antimicrob Agents Chemother. 2017;61(3). doi:10.1128/aac.02319-16
44. Abdelraouf K, Braggs KH, Yin T, et al. Characterization of polymyxin B-induced nephrotoxicity: implications for dosing regimen design. Antimicrob Agents Chemother. 2012;56(9):4625–4629. doi:10.1128/aac.00280-12
45. Sisay M, Hagos B, Edessa D, et al. Polymyxin-induced nephrotoxicity and its predictors: a systematic review and meta-analysis of studies conducted using RIFLE criteria of acute kidney injury. Pharmacol Res. 2021;163:105328. doi:10.1016/j.phrs.2020.105328
46. Xu Y, Liang P, Liu N, et al. Correlation between the drug concentration of polymyxin B and polymyxin B-associated acute kidney injury in critically ill patients: a prospective study. Pharmacol Res Perspect. 2022;10(5):e01010. doi:10.1002/prp2.1010
47. Han L, Xu FM, Zhang XS, et al. Trough polymyxin B plasma concentration is an independent risk factor for its nephrotoxicity. Br. J. Clin. Pharmacol. 2022;88(3):1202–1210. doi:10.1111/bcp.15061
48. Mattos KP, Lloret GR, Cintra ML, et al. Acquired skin hyperpigmentation following intravenous polymyxin B treatment: a cohort study. Pigm Cell Mel Res. 2016;29(3):388–390. doi:10.1111/pcmr.12468
49. Mattos KPH, Cintra ML, Gouvêa IR, et al. Skin hyperpigmentation following intravenous polymyxin B treatment associated with melanocyte activation and inflammatory process. J Clin Pharm Therapeutics. 2017;42(5):573–578. doi:10.1111/jcpt.12543
50. Liu J, Shao M, Xu Q, et al. Low-dose intravenous plus inhaled versus intravenous polymyxin B for the treatment of extensive drug-resistant Gram-negative ventilator-associated pneumonia in the critical illnesses: a multi-center matched case-control study. Ann Intens Care. 2022;12(1):72. doi:10.1186/s13613-022-01033-5
51. Gothwal S, Meena K, Sharma SD. Polymyxin B induced generalized hyperpigmentation in neonates. Indian J Pediatr. 2016;83(2):179–180. doi:10.1007/s12098-015-1798-z