Fitness
Simultaneous Detection of Helicobacter pylori | IDR
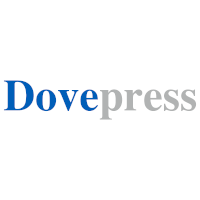
Introduction
Helicobacter pylori (H. pylori), a Gram-negative microaerobic flagellated bacterium, impacts a minimum of 50% of the worldwide population based on data from the World Gastrointestinal Organization (WGO).1 According to a study conducted in China, the prevalence of H. pylori infection among the general population was found to be 40.66%.2 H. pylori causes chronic gastritis, which can lead to severe gastroduodenal conditions such as peptic ulcers and gastric cancer.3–5 In addition, H. pylori infection is widely believed to be the primary etiological factor in approximately 90% of gastric cancer cases,6,7 and the World Health Organization (WHO) and the International Agency for Research on Cancer (IARC) have classified it as a class I carcinogen.8 The initial step towards the treatment and elimination of infections caused by microorganisms is the precise diagnosis. 13/14C-urea breath test (13/14C-UBT) and endoscopic gastric mucosal biopsy are commonly used clinical examination,4,9 besides, some researchers have developed Quantitative Real-time Polymerase Chain Reaction(qPCR) based techniques for H. pylori detection using saliva or stool specimens.10,11 However, these methods require skilled practitioners and specialized equipment, making them impractical for grassroots institutions. Fecal antigen detection serves as a representative non-invasive examination, has the advantages of being economical and simple; while its sensitivity remains a limiting factor.9 To effectively reduce the spread of this disease, there is an urgent need for a rapid, easy-read, and highly sensitive detection approach that can be conveniently employed for pre-hospital detection and mass health screening.
According to a recent study, the prevalence of clarithromycin resistance in H. pylori in China has escalated to 34%.12 This resistance is primarily attributed to the presence of nucleotide mutations A2143G and A2142G in the V domain of 23S rRNA.9,13 The restricted selection of efficacious antibiotics, coupled with the improper utilization and overuse of antibiotics, has led to an escalation in H. pylori resistance and ineffective treatment.12 Hence, there is a pressing need for a streamlined, highly sensitive, and specific detection method to identify clindamycin resistance, thereby providing treatment strategies and adjusting medication promptly. The drug sensitivity test following bacterial culture is widely regarded as the definitive method for detecting drug resistance in clinical practice,14 however, the procedure is laborious, time-intensive, and yields a low rate of success. For the detection of resistance genes, researchers have developed qPCR-based method for simultaneous detection of H. pylori and antimicrobial resistance with high sensitivity and specificity.9,15
In recent years, clustered regularly interspaced short palindromic repeats (CRISPR) based nucleic acid detection technology has been considered as the next-generation molecular diagnostics.16,17 Specific High-Sensitivity Enzymatic Reporter UnLOCKing (SHERLOCK), developed by Gootenberg et al, is a CRISPR/Cas13a-based powerful, scalable diagnostic technology to detect pathogen-specific nucleic acid and distinguish single nucleotide mutations.18,19 We have previously developed the easy-readout and sensitive enhanced (ERASE) assay, which combines CRISPR and lateral flow test strip to detect COVID-19 with a sensitivity of 1 copy/μL.20 In the study, we have developed the sensitive H. pylori identified easy-read dual detection (SHIELD) assay for H. pylori detection, which enables simultaneous detection of H. pylori and clarithromycin resistance mutations on a single lateral flow strip. This method offers enhanced ease of operation, time efficiency, and eliminates the need for costly and specialized equipment, and provides a new measure for monitoring the occurrence and development of H. pylori infection and guidance on the administration of clinical medication.
Methods
Saliva Sample Collection and DNA Extraction
Saliva samples were obtained from a cohort of 28 patients at the Sixth Medical Center of Chinese PLA General Hospital. All patients included had gastrointestinal symptoms of epigastric pain, belching and acid reflux. Inclusion criteria for sample collection: patients between 18 and 70 years of age; patients have no other underlying disease; samples collected, stored and processed in accordance with reagent requirements. Following the rinsing of the oral cavity, a minimum waiting period of 10 minutes is advised. Subsequently, the patient should be instructed to allow saliva to naturally flow into the collection tube, ensuring the collection of 2mL. Combine an equal volume of 2mL preservation solution with the sample, ensuring thorough mixing by inverting the mixture. Finally, the collected samples were stored at a temperature range of 2–8 °C. DNA extraction steps were performed executed in accordance with the instructions provided by the saliva DNA collection and extraction kit (Genenode, China).
Nucleic Acid Preparation
The protocols preparation for nucleic acid are provided in the Supplementary Materials and Methods.
Quantitative Real-Time PCR (qPCR) Assay
The qPCR detection system consisted of 20 μL, including 10 μL TaqMan™ Fast Advanced Master Mix (Thermo Scientific, USA), 0.5 μL forward primers (10µM), 0.5 μL reverse primers (10µM), 0.5 μL qPCR probes (10µM), 5.5 μL RNase free water (TaKaRa, Japan), 3 μL template DNA. The qPCR assay was conducted using the subsequent thermal cycling protocol: pre-denaturation at 95°C for 10 min, followed by 40 cycles consisting of denaturation at 95°C for 15s and annealing at 60°C for 45s.
RAA Fluorescence Detection System
RAA fluorescence detection were performed using RAA kit from ZC Biotechnology Co., Ltd. (Hangzhou, China).The system consisted of 50 μL: add 25 μL A Buffer, 2.0 μL forward primers (10µM), 2.0 μL reverse primers (10µM), 0.6 μL EXO-probes (10µM), 7.9 μL RNase free water (TaKaRa, Japan) and 10.0 μL template DNA were combined into the detection unit tube containing dry reaction powder; 2.5 μL Buffer B was then added to the detection unit’s tube cover, and the mixture was well mixed with gentle shaking upside down and rapidly centrifuged at low speed. The reaction mixture was then placed in a CFX 96 Touch (Bio-Rad, USA), the reactions were incubated for 15 min at 39 °C and fluorescence intensity was detected with excitation light of 495 nm and emission at 520 nm once every 30 seconds.
RAA-CRISPR-Cas13a Fluorescence Detection System
RAA reactions were performed using RAA kit from ZC Biotechnology Co., Ltd. (Hangzhou, China). The system consisted of 25 μL of Buffer A, 2.0 μL forward primers (10µM), 2.0 μL reverse primers (10µM), 8.5 μL of RNase-free water and 10.0 μL template DNA were combined into the detection unit tube containing dry reaction powder; 2.5 μL Buffer B was then added to the detection unit’s tube cover, and the mixture was well mixed with gentle shaking upside down and rapidly centrifuged at low speed. The reactions were incubated at 39°C for 30 min. After amplification, 5 μL of the RAA product was analyzed using Cas13a-based detection.
The experimental protocol involved the utilization of the subsequent constituents: 2 μL NTP (New England Biolabs, USA), 1 μL of RNase inhibitor (New England Biolabs, USA), 45 nM LwCas13a (GenScript, China), 20 mM HEPES buffer solution (Thermo Scientific, USA), 10 mM MgCl2 (Thermo Scientific, USA) solution, 1.5 μL crRNA, 0.5 μL of T7 RNA Polymerase (NEB, USA), 2.5 μL reporter RNA (Thermo Scientific, USA), 5 μL of RAA amplified product, and 10.75 μL of RNase-free water. The reactions were carried out at 37°C for 60 min using CFX Opus 96 (Bio-Rad, USA) every 2 min.
The SHIELD Assay
The SHIELD assay consists of two parts. The system of RAA reactions using labeled primers consist of 25 μL of Buffer A, 2.0 μL forward primers (1.6 µM), 2.0 μL labeled forward primers (0.4 µM), 2.0 μL labeled reverse primers (2 µM), 6.5 μL of RNase-free water and 10.0 μL template DNA and 2.5 μL Buffer B. The reactions were incubated at 39°C for 30 min. After amplification, 5 μL of the RAA product was analyzed using Cas13a-based detection.
The experimental protocol involved the utilization of the subsequent constituents: 4 μL NTP (New England Biolabs, USA), 2 μL of RNase inhibitor (New England Biolabs, USA), 45 nM LwCas13a (GenScript, China), 20 mM HEPES buffer solution (Thermo Scientific, USA), 10 mM MgCl2 solution, 3 μL crRNA, 1 μL of T7 RNA Polymerase (NEB, USA), 5 μL reporter RNA (RNase Alert, Thermo Scientific, USA), 5 μL of RAA amplified product, and 26.5 μL of RNase-free water. The reactions were incubated at 37°C for 30 min. Then add the reaction products onto the strip, observe the results in 3–10 minutes.
Lateral Flow Strip Detection Result Readout
The control line (C) is situated at the uppermost part of the membrane, while the test line 1 (T1) was at the bottom of the membrane, with test line 2 (T2) situated between these two lines. The distance separating each line measured 5 mm. 0.5 mg/mL of Digoxin antibody (Jtests Science, China), 0.5 mg/mL of streptavidin (Seebiotech, China) and 1.5 mg/mL of goat antirabbit Immunoglobulin G (Sangon Biotech, China) were sprayed onto nitrocellulose membrane (Sartorius Stedim, Germany) to form the T1, T2 and C lines. When strips were observed on both the C line and the two T lines, the interpretation of the results indicated positive and wild type (means the strain is H. pylori with no resistance). When bands were observed on the C line and T1 line of the test strip while T2 line was unobservable, the findings were construed as positive and mutant (means the strain is H. pylori with clarithromycin resistance mutations). The observation solely encompassed the presence of C line and T2 line, indicating negative result (means the strain is not H. pylori).
Statistical Analysis
The H. pylori DNA results are expressed as copies/µL (template concentration). Data were analyzed by GraphPad Prism 8.0.2 software (GraphPad, Inc., La Jolla, CA, USA). Mean differences in the data were determined by paired t-test, two-way ANOVA and Fisher’s exact test. The data are represented as the mean ± SEM. All statistical tests are two-sided, statistical significance was defined as P
Results
Design of SHIELD Detection Method for H. Pylori
As shown in Figure 1, the patient’s saliva samples were collected and processed to extract nucleic acids. Then, in the amplification step, the 5’ end of the forward and reverse primers were respectively modified with FAM and digoxin. In addition, the T7 promoter sequence was added ahead the forward primer, to enable the T7 RNA polymerase to bind and transcribe ssRNA in the detection step. Following the process of RAA amplification, it is possible to generate double-stranded DNA (dsDNA) with FAM attached at the 5’-end and digoxin (DIG) attached at the 3’-end. Then the corresponding DIG antibody and streptavidin were sprayed on the strip to form the test line 1 and test line 2. And under the guidance of crRNA, Cas13a protein recognizes and binds to the target RNA sequence, subsequently, the non-specific cleavage ability of Cas13a protein to ssRNA was activated, reporter RNA labeled with FAM and Biotin could be cut off, so the band of this test line T2 would be eliminated. After adding the product to the test strip, we can observe the results with the naked eye. That is, detecting H. pylori infection by isothermal amplification and detecting resistant mutations by CRISPR technology.
Screening RAA Primers for H. Pylori Detection
To develop a detection method for H. pylori based on RAA, sequence alignment was first conducted. A sequence of the target 23S rRNA gene (350 bp) was selected and constructed into a plasmid, 5 pairs of RAA primers and an RAA-EXO-probe were designed according to the sequence with over 90% conserved (Figure 2A and Table S1). Fluorescence detection was performed using all pairwise combinations of forward and reverse primers to amplify plasmids at a concentration of 103 copies/μL. The result show that primer combination F3R3 had the highest RFU at 10min, seemed as optimal amplification efficiency (Figure 2B). And the Agarose gel electrophoresis of the RAA products also showed expected bands (169bp) (Figure S1). So, this pair of primers were used for further study. To determine the sensitivity of the detection system, then gradient diluted H. pylori plasmid was used for RAA fluorescence detection. The fluorescence value of the experimental group “10° copies/μL” was 338 ± 70.84 a.u. at 20min (Figure 2C and D), a statistically significant difference (P H. pylori by this method is 1 copy/μL, with a corresponding Ct Value of 23.03 ± 1.67 (Figure S2). The qPCR detection was possible to detect a minimum plasmid concentration of 10° copies/μL, with a corresponding Ct Value of 37.13 ± 0.72 (Figure 2E). Moreover, to evaluate the specificity of RAA detection of H. pylori, DNAs of 5 kinds of common bacteria in alimentary canal were used for the fluorescence detection, the results showed that the method had no cross-reaction with the 5 bacteria, and the method showed good specificity with a statistically significant difference (P Figure 2F).
Screening Efficient crRNA for Mutation-Detection
Many researchers have reported that the single base mutation of H. pylori 23S rRNA gene leads to clarithromycin resistance, majority of them occurred at 2142 and 2143 sites, thus, we synthesized these two mutant type plasmids. Moreover, when detecting wild type, some reported show that introduction of single base mutation has little effect on detection of mutant strains, but two consecutive single base mutations probably have an impact on detection. Thus, we designed 4 crRNAs (Figure 3A), converted A to G both at position 2142 and 2143 for the purpose of detect these 2 kinds of mutant strains using one crRNA. Then screening of four crRNAs using plasmids was performed with the CRISPR‒Cas13a fluorescence assay. As shown in Figure 3B and C, the fluorescence value of crRNA4 reached 28175.33 ± 1765.14 a.u. for A2142G plasmid and 46867.01 ± 5734.79 a.u. for A2143G plasmid at 30 min. crRNA4 was effective in detecting both mutant plasmids, showed the most remarkable specificity (P0.05). To determine the sensitivity of the detection system, gradient diluted 2 mutation plasmids and 1 wild plasmid were used for CRISPR detection. The fluorescence values of 4 four experimental groups (104 copies/μL ~101 copies/μL) were significantly increased at 30 min (P1 copies/μL” reached 7781.67 ± 448.72 a.u. for A2142G plasmid and 9216.33 ± 477.50 a.u. for A2143G plasmid, showed the LoD of detecting 2 mutation plasmids were both 101 copies/μL (Figure 3D). Normally, clinical infections are not purebred, so we mixed the mutant plasmid and wild plasmid in different proportions with concentration of 5×103 copies/μL, to simulate H. pylori heterozygotes. The result showed the detection system could detect mixed samples as low as 5% (Figure 3E).
Evaluation of SHIELD Detection Methods
To present the detection results rapidly and conveniently, we combined the above mentioned two detection methods with lateral flow test strips, called SHIELD. Add the liquid onto the sample pad, then it will sequentially flow through colloidal gold bonding pad (colloidal gold labeled with FAM antibody), test line 1 (T1, Digoxin antibody), test line 2 (T2, Streptavidin), control line and absorption pad. T1 will remain the colloidal gold molecules through labeled target DNA at both ends (Digoxin-target DNA-FAM). Target nucleotide will activate Cas13a to cleave the reporter RNA (Biotin-reporter RNA -FAM), T2 will not remain the colloidal gold molecules. So that we could diagnosis H. pylori infection by T1 and drug-resistant strain by T2 (Figure 4A). We added various elements onto strips to assess its usability. The obtained experimental findings align with the anticipated outcomes (Figure 4B). To mitigate the impact of primer dimer on the detection process, we additionally optimized the concentration of labeled primers (Figure S3). The method was verified using 10-fold gradient dilution of plasmids. The test paper results for WT plasmid indicated a positive and no drug resistant outcome when the plasmid concentration exceeded 5×101 copies/μL, while the results for A2142G plasmid and A2143G plasmid indicated a positive and drug resistant outcome when the plasmid concentration exceeded 5×101 copies/μL. Thus, the LoD of the detection method were 5×101 copies/μL (Figure 4C).
Saliva Samples Were Tested Using the SHIELD Assay
To evaluate the efficacy of this method in clinical samples, we selected the saliva of clinical patients as the sample to be tested. Twenty-eight saliva samples were collected from patients to verify the practicability of the detection method. DNA extracted from saliva was also subjected to qPCR and 23S rRNA gene sequencing (Figure 5). In comparison to the qPCR findings, the detection outcomes of the established method exhibited complete congruity at a rate of 100% (Table 1A). In comparison to the gene sequencing results, 26 samples were found to be identical; 2 samples were tested mutation by gene sequencing but wild by SHIELD. Referred to the results of qPCR and gene sequencing, we considered those 2 samples as heterozygous with low DNA concentrations (Figure 5 and Table 1B). Compared with C13/14-UBT, the SHIELD method successfully identifies 17 out of the 22 UBT-positive samples, and accurately distinguishes 9 of them as drug-resistant mutants (Table S2).
![]() |
Figure 5 The detection of saliva samples. 28 saliva samples were detected using qPCR, gene sequencing and SHIELD assay. |
Discussion
H. pylori detection is a prerequisite for treating and interrupting the spread of the disease, and researchers have developed a range of detection methods. Gastric mucosal biopsy allows direct detection of pathogens and is often considered the gold standard test, while it is generally not recommended as a first choice due to cost and patient tolerability.21 C13/14-UBT is widely used in medical examinations and outpatient clinics because of its specificity and sensitivity of more than 95%, however, drug resistance cannot be detected by the method.9,22 Molecular methods like gene sequencing and PCR-based detection are capable of detecting infections and drug-resistant, these methods are used in clinical applications because of the high sensitivity and low risk of cross-infection.23,24 In this study, we developed a simultaneous detection method to identify H. pylori and clarithromycin resistance mutations based on RAA-CRISPR system. The RAA amplification step serves a dual purpose, as it not only amplified the target DNA fragment but also employs the resulting amplified product for the identification of pathogen infection; CRISPR-Cas13a detection system is utilized for the detection of drug resistance. Ultimately, the results were readout through the lateral flow test strip. The detection of synthetic plasmids and saliva samples indicated that the SHIELD assay has high sensitivity and specificity and is capable of delivering precise and convenient results that could be read with the naked eye, offers technical assistance for future implementation of point-of-care testing and large-scale screening. Its implementation can help mitigate familial and clustered transmission, decrease the population’s infection rate, and prevent ineffective medication in the future.
Further, this method could distinguish the most common mutations A2142G and 2143G that cause clarithromycin resistance while detecting H. pylori. The resistance rates of A2142G and A2143G in various studies were found to be 16–17% and 80–90% for clinical isolates, respectively.3 Detection of drug resistance of infected strains can also guide clinical medication, avoid the abuse and ineffective use of antibiotics, improve the success rate of eradication therapy. For other drug resistance can also be detected by this method, only need to change the primers and crRNA. The results are presented using test strips without the need for professional personnel and laboratories, it is suitable for patient self-monitoring and treatment effect evaluation. The temperature required for the reaction is 37 °C~39 °C, the whole detection process does not require precise instruments, only a small heating device is needed. The simplicity, good specificity and sensitivity provide the possibility for large-scale screening and on-site testing. Given the advancements and progress in bacterial nucleic acid extraction technology, such as direct boiling, mechanical lysis,25,26 the integration of SHIELD assay with streamlined and rapid nucleic acid extraction techniques needs further research.
Several studies have indicated the presence of H. pylori in saliva and dental plaque, which correlates with the colonization of H. pylori in the gastric mucosa.27–29 The collection of saliva is notably more convenient when compared to obtaining gastric mucosa, blood, and fecal samples. Consequently, we have opted to utilize saliva as chosen sample. This method may also applicable to other nucleic acid samples such as gastric mucosa and feces, because the CRISPR system has strong anti-interference. The observed discrepancy between the established detection method and the results of C13/14-UBT may be attributed to the limited sample size, besides, potential factors contributing to this inconsistency include sampling errors and variations in bacterial colonization locations. In subsequent studies, we will continue to collect samples to evaluate the method. Additionally, the concentration of nucleic acid in saliva is relatively low and susceptible to degradation by other enzymes.
Ethics Approval
The study protocol satisfied the ethical guidelines of the 1975 Declaration of Helsinki and was approved by the medical ethics committee of Chinese People’s Liberation Army General Hospital (protocol code s2022-38). All donors provided informed consent.
Author Contributions
All authors made a significant contribution to the work reported, whether that is in the conception, study design, execution, acquisition of data, analysis and interpretation, or in all these areas; took part in drafting, revising or critically reviewing the article; gave final approval of the version to be published; have agreed on the journal to which the article has been submitted; and agree to be accountable for all aspects of the work.
Funding
This study was supported by the National Key Research and Development Program of China [grant numbers 2023YFC2605100, 2021YFC2301100].
Disclosure
Professor Yansong Sun reports a pending patent RAA/CRISPR-based dual-target detection method. The authors report no other conflicts of interest in this work.
References
1. Malfertheiner P, Camargo MC, El-Omar E, et al. Helicobacter pylori infection. Nat Rev Dis Primers. 2023;9(1):19. doi:10.1038/s41572-023-00431-8
2. Zhou XZ, Lyu NH, Zhu HY, et al. Large-scale, national, family-based epidemiological study on Helicobacter pylori infection in China: the time to change practice for related disease prevention. Gut. 2023;72(5):855–869. doi:10.1136/gutjnl-2022-328965
3. Camilo V, Sugiyama T, Touati E. Pathogenesis of Helicobacter pylori infection. Helicobacter. 2017;22(Suppl 1):1. doi:10.1111/hel.12405
4. Sugano K, Tack J, Kuipers EJ, et al. Kyoto global consensus report on Helicobacter pylori gastritis. Gut. 2015;64(9):1353–1367. doi:10.1136/gutjnl-2015-309252
5. Peek RJ, Blaser MJ. Helicobacter pylori and gastrointestinal tract adenocarcinomas. Nat Rev Cancer. 2002;2(1):28–37. doi:10.1038/nrc703
6. Hooi J, Lai WY, Ng WK, et al. Global prevalence of Helicobacter pylori infection: systematic review and meta-analysis. Gastroenterology. 2017;153(2):420–429. doi:10.1053/j.gastro.2017.04.022
7. Lee DG, Kim HS, Lee YS, et al. Helicobacter pylori CagA promotes Snail-mediated epithelial-mesenchymal transition by reducing GSK-3 activity. Nat Commun. 2014;5:4423. doi:10.1038/ncomms5423
8. Iarc L. Schistosomes, liver flukes and Helicobacter pylori. IARC Monogr Eval Carcinog Risks Hum. 1994;61:1–241.
9. Ansari S, Yamaoka Y. Helicobacter pylori infection, its laboratory diagnosis, and antimicrobial resistance: a perspective of clinical relevance. Clin Microbiol Rev. 2022;35(3):e0025821. doi:10.1128/cmr.00258-21
10. Rimbara E, Sasatsu M, Graham DY. PCR detection of Helicobacter pylori in clinical samples. Methods Mol Biol. 2013;943:279–287.
11. Duś I, Dobosz T, Manzin A, et al. Role of PCR in Helicobacter pylori diagnostics and research – new approaches for study of coccoid and spiral forms of the bacteria. Postepy Hig Med Dosw. 2013;67:261–268. doi:10.5604/17322693.1044005
12. Chen J, Li P, Huang Y, et al. Primary antibiotic resistance of Helicobacter pylori in different regions of china: a systematic review and meta-analysis. Pathogens. 2022;11(7):786. doi:10.3390/pathogens11070786
13. Chen D, Cunningham SA, Cole NC, et al. Phenotypic and molecular antimicrobial susceptibility of Helicobacter pylori. Antimicrob Agents Chemother. 2017;61(4). doi:10.1128/AAC.02530-16
14. Talebi BAA. Diagnosis of Helicobacter pylori using invasive and noninvasive approaches. J Pathog. 2018;2018:9064952. doi:10.1155/2018/9064952
15. Gazi S, Karameris A, Christoforou M, et al. Real-Time PCR detection and quantitation of Helicobacter pylori clarithromycin-resistant strains in archival material and correlation with Sydney classification. Ann Gastroenterol. 2013;26(3):226–232.
16. Kaminski MM, Abudayyeh OO, Gootenberg JS, Zhang F, Collins JJ. CRISPR-based diagnostics. Nat Biomed Eng. 2021;5(7):643–656. doi:10.1038/s41551-021-00760-7
17. Wang X, Shang X, Huang X. Next-generation pathogen diagnosis with CRISPR/Cas-based detection methods. Emerg Microbes Infect. 2020;9(1):1682–1691. doi:10.1080/22221751.2020.1793689
18. Gootenberg JS, Abudayyeh OO, Lee JW, et al. Nucleic acid detection with CRISPR-Cas13a/C2c2. Science. 2017;356(6336):438–442. doi:10.1126/science.aam9321
19. Wang S, Li H, Kou Z, et al. Highly sensitive and specific detection of hepatitis B virus DNA and drug resistance mutations utilizing the PCR-based CRISPR-Cas13a system. Clin Microbiol Infect. 2021;27(3):443–450. doi:10.1016/j.cmi.2020.04.018
20. Li H, Dong X, Wang Y, et al. Sensitive and easy-read CRISPR Strip for COVID-19 rapid point-of-care testing. CRISPR J. 2021;4(3):392–399. doi:10.1089/crispr.2020.0138
21. Lee JY, Kim N. Diagnosis of Helicobacter pylori by invasive test: histology. Ann Transl Med. 2015;3(1):10. doi:10.3978/j.issn.2305-5839.2014.11.03
22. Leal YA, Flores LL, Fuentes-Pananá EM, Cedillo-Rivera R, Torres J. 13C-urea breath test for the diagnosis of Helicobacter pylori infection in children: a systematic review and meta-analysis. Helicobacter. 2011;16(4):327–337. doi:10.1111/j.1523-5378.2011.00863.x
23. Gong RJ, Xu CX, Li H, Liu XM. Polymerase chain reaction-based tests for detecting Helicobacter pylori clarithromycin resistance in stool samples: a meta-analysis. World J Clin Cases. 2021;9(1):133–147. doi:10.12998/wjcc.v9.i1.133
24. Mannion A, Dzink-Fox J, Shen Z, et al. Helicobacter pylori antimicrobial resistance and gene variants in high- and low-gastric-cancer-risk populations. J Clin Microbiol. 2021;59(5). doi:10.1128/JCM.03203-20
25. Rosenbaum J, Usyk M, Chen Z, et al. Evaluation of oral cavity DNA extraction methods on bacterial and fungal microbiota. Sci Rep. 2019;9(1):1531. doi:10.1038/s41598-018-38049-6
26. Li S, Liu X, Li Z, Liu H, Hu D. Combination of direct boiling and glass beads increases the purity and accuracy of bacterial DNA extraction. Biotechnol J. 2023;18(11):e2300135. doi:10.1002/biot.202300135
27. Brown LM. Helicobacter pylori: epidemiology and routes of transmission. Epidemiol Rev. 2000;22(2):283–297. doi:10.1093/oxfordjournals.epirev.a018040
28. Anand PS, Kamath KP, Anil S. Role of dental plaque, saliva and periodontal disease in Helicobacter pylori infection. World J Gastroenterol. 2014;20(19):5639–5653. doi:10.3748/wjg.v20.i19.5639
29. Mao X, Jakubovics NS, Bächle M, et al. Colonization of Helicobacter pylori in the oral cavity – an endless controversy? Crit Rev Microbiol. 2021;47(5):612–629. doi:10.1080/1040841X.2021.1907740