Tech
Stereotactic Body Radiation Therapy for LAPC | CEG
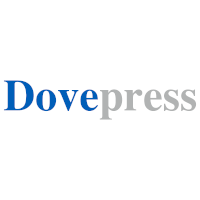
Introduction
Pancreatic ductal adenocarcinoma (PDAC) was estimated to affect more than 62,000 patients in 2022.1 Margin-negative surgical resection is generally considered the only curative treatment for PDAC; however, due to the infiltrating nature of the disease and relatively high proportion of patients who have distant metastasis at initial diagnosis, only 20% are considered suitable for an up-front curative-intent operation. Furthermore, approximately 30% have locally advanced PDAC (LAPC), a highly heterogeneous disease entity, anatomically characterized by locoregionally confined disease but extensive arterial and/or venous involvement generally precluding an operation.2 There is a need for innovation and investigation to advance outcomes for this population.
American Society of Clinical Oncology (ASCO) and American Society for Radiation Oncology (ASTRO) consensus guidelines suggest that there is limited evidence to support just one optimal treatment regimen for patients with LAPC; patients should be offered trial participation.3,4 Both organizations suggest that treatment for most patients should begin with systemic multiagent chemotherapy such as fluorouracil, leucovorin, irinotecan and oxaliplatin (FOLFIRINOX) or gemcitabine and nanoparticle albumin-bound paclitaxel (Gem/nab-Paclitaxel). After systemic therapy, there are conditional recommendations for radiation therapy (RT) in patients who do not develop metastatic disease.3,4 The lack of consensus management stems from the nature of PDAC as a highly heterogeneous disease, competing evidence from large randomized clinical trials and dynamic changes in the treatment landscape.5–8 Taking that into consideration, current evidence suggests that RT can improve locoregional control, extend the systemic treatment-free interval, aid in the treatment and/or selection of potential operative candidates, and palliate local symptoms.5,9,10 More recently, data has suggested favorable local control and overall survival (OS) in well-selected patients treated with RT to high biologically effective doses (BED), compared with historical series utilizing conventional RT dose and fractionation schemes of 45–54 Gy in 1.8–2.0 Gy fractions.11–13
Stereotactic body RT (SBRT) is a promising RT technique which leverages a combination of highly precise patient immobilization, accurate image guidance, and respiratory motion management techniques to deliver a highly conformal-dose distribution, enabling the safe delivery of higher doses per fraction while minimizing dose to adjacent structures. SBRT is delivered in 5 fractions or less, over 1–2 weeks, thus providing the added advantage of improved patient convenience and less time off of systemic therapy.14
In this review, we present a historical perspective on the role of SBRT for LAPC, outline advances in SBRT delivery and present a framework for selecting patients for treatment with SBRT.
Conventionally Fractionated (Chemo)Radiation for LAPC
Early clinical trials evaluating the role of conventionally fractionated chemoradiation (CRT) for LAPC suggested improved OS with the use of RT.6,7,15 For example, the early Gastrointestinal Tumor Study Group (GITSG) trials, which utilized a range of 40–60 Gy split course RT regimens, suggested improved OS with the use of CRT vs RT alone or chemotherapy alone.7,8 Similarly, the Eastern Cooperative Oncology Group 4201 trial found a modest, 2-month, median OS benefit for patients treated with CRT vs gemcitabine-based chemotherapy.16 Despite these improvements in OS, most patients developed distant metastasis and ultimately died from PDAC.
The relevance of these findings in contemporary clinical practice is debatable, however, as current practice generally involves the use of initial systemic therapy and interval re-assessment to help select for patients with a more favorable disease biology who may then benefit from locally consolidative RT.5,17 The initial The Groupe Coopérateur Multidisciplinaire en Oncologie (GERCOR) trials supported this hypothesis and ultimately led to the development of the LAP07 trial, which randomized patients who did not progress after an initial 4-months of gemcitabine-based chemotherapy to chemotherapy alone vs the addition of capecitabine-based CRT to a dose of 54 Gy in 30 fractions.5,18 CRT was shown to be associated with improved locoregional control and prolonged systemic therapy-free interval, but no improvement in OS.
It is notable, however, that even after careful “selection” of patients using an initial phase of gemcitabine chemotherapy, 44–60% experienced distant metastatic progression. This led many to hypothesize that if systemic therapy could be further optimized to gain better control of gross and/or occult distant disease, then the relative competing risks of locoregional progression would increase and thereby further support the role of locoregional therapies.
In 2011, Conroy et al demonstrated that FOLFIRINOX was associated with improved objective response and OS when compared with gemcitabine-alone for patients with metastatic PDAC.19 Similarly, von Hoff et al demonstrated improved objective response and OS with the use of Gem/nab-Paclitaxel compared with gemcitabine alone.20 These regimens were then incorporated into the care of patients with LAPC and more recently have been demonstrated to improve PFS, objective response, and OS, when compared to gemcitabine alone, amongst patients with locoregional disease.21–23
For patients with LAPC, the Chemoradiation Compared With Chemotherapy Alone After Induction Chemotherapy (CONKO-007) trial utilized a regimen of initial FOLFIRINOX (77%) or gemcitabine (23%) and subsequently randomized patients who did not have interval progression to continued chemotherapy or consolidative gemcitabine-based conventionally fractioned CRT (50.4 Gy in 28 fractions).24 CRT was not associated with improved OS in the overall cohort; however, it was associated with a higher rate of R0 resection (50% versus 69%) in patients who ultimately underwent a curative-intent operation. R0 resection and R0 circumferential resection margin of greater than 1 mm were independently associated with improved survival. Subset analysis of the patients who proceeded with surgical resection demonstrated that CRT was associated with notable improvement in 5-year survival, 13% versus 27%. Improvements in these surgical surrogate outcomes, which are associated with survival, support consideration of consolidative CRT in patients who do not progress after intensive induction chemotherapy.
Evolving Role of SBRT for LAPC
Contemporary application of SBRT or dose-escalated fractionated RT for this population provide benefits over the LAP07 and CONKO-007 approaches. Improvements in patient immobilization, respiratory motion management, image guidance, and ultra-conformal radiotherapy delivery with intensity modulated RT or SBRT have enabled the safe delivery of higher total doses and dose per fraction of radiation, which are associated with improved local control while maintaining safety. SBRT also has the benefit of improved patient convenience and decreasing time off of systemic therapy by compressing the RT regimen from what used to be 5–6 weeks of daily RT to = 5 fractions over 1–2 weeks. Appreciation of the current role of SBRT for patients with LAPC, necessitates reflecting on the historical experiences, evolution in systemic therapy, and advancements in treatment delivery.
Early SBRT Experience
Koong et al pioneered the initial studies of SBRT in PDAC. Their initial Phase I trial, which utilized a regimen of 25 Gy in 1 fraction, garnered much enthusiasm for the possible applications of SBRT in treating various GI malignancies.25 The study included 6 patients and at relatively short follow-up of 4.5 months; there was an early signal of efficacy with 100% local control. Late toxicities that became evident with further follow-up and in subsequent trials ultimately tempered enthusiasm for single fraction SBRT.26–28
Early investigation of fractioned SBRT for LAPC was conducted by Hoyer et al, who treated 22 patients with 45 Gy in 3 fractions.29 Delivering a lower dose per fraction, but higher overall dose, was thought to improve toxicity rates (particularly late toxicity) without sacrificing local control. However, with several local failures and unacceptable toxicity, the authors concluded that SBRT was not feasible for LAPC. This early skepticism about implementing SBRT was likely colored by the limitations with intrafraction motion management and a more limited understanding of dose-volume predictors of toxicity for organs at risk at that time.
Despite the initial suboptimal results, the early trials and cohorts of patients treated with SBRT for LAPC developed a strong foundation of data to inform our understanding of PDAC dose response and tolerance of organs at risk with respect to acute and late toxicity.26,30 Mahadevan et al built upon these data and reported a risk-adapted approach to delivering SBRT for LAPC.31,32 They delivered 24 Gy in 3 fractions to tumors abutting one-third or more of the duodenum, 30 Gy in 3 fractions to tumors abutting the bowel in only one area, and 36 Gy in 3 fractions to tumors ≥3 mm from the duodenum. They found that this approach (with or without induction chemotherapy) was safe with reasonable efficacy compared to the contemporary data of conventional chemoradiotherapy.
A meta-analysis reported a pooled percentage of locoregional control at 1 year after SBRT of 72% (95% confidence interval: 59–79%).33 This estimate, along with other non-randomized studies, demonstrated comparable locoregional control in patients treated with conventionally fractionated IMRT and SBRT.7,12,34–37 Additionally, one meta-analysis presented by Tchelebi et al demonstrated superior 2-year overall survival for patients receiving SBRT vs CRT (26.9% vs 13.7%) and lower risk of grade 3/4 toxicity (5.6% vs 37.7%).37 These results, however, should be interpreted with caution because it is likely that selection criteria for SBRT trials favored patients with lower volume of disease and fewer involved lymph nodes.
There has been a growing body of evidence supporting the use of SBRT and further guiding us on dose-response and organ-at-risk dose-volume thresholds. Select prospective studies of SBRT from 2015 to 2023 are presented in Table 1.
Table 1 Select Prospective Studies of Stereotactic Body Radiation Therapy for Locally Advanced Pancreatic Cancer, 2015–2023 |
SBRT Following Induction Chemotherapy
While there is still limited evidence to support the widespread use of RT, including SBRT, for patients with LAPC, there is a consensus that multiagent chemotherapy should precede locoregional treatment with RT for the majority of patients.3,4 Induction chemotherapy allows for systemic disease control, tumor downstaging, and selection of patients who might have a disease phenotype that may benefit from locoregional treatment (eg, those who do not develop early metastatic disease). Some of the first series of SBRT for LAPC assessed upfront chemotherapy before selecting patients for local therapy.32,38 These initial studies found that 17–30% of patients who are locally advanced at enrollment end up developing metastases while on chemotherapy before receiving SBRT, matching the estimate (29.3%) from the GERCOR Phase II and III studies.17 These patients are unlikely to have benefitted from locoregional treatment given their distant progression while on systemic therapy. Induction gemcitabine prior to SBRT was also assessed in a Phase II trial of 60 patients (49 patients analyzed).41 The investigators did not explicitly require restaging after 3 weeks of gemcitabine, but 2 (4.1%) patients were excluded due to “rapid tumor progression”. One would expect that with planned restaging, more patients would have been found to have progressed.
While the initial results with induction gemcitabine were promising, there were other contemporary trials showing the superiority of multiagent chemotherapy in the metastatic setting.19,20 With these data, practice patterns and prospective studies began to shift in the LAPC setting, favoring aggressive induction chemotherapy before consideration of RT.13,40,42–45 Importantly, intensification of chemotherapy was also paired with dose escalated SBRT, confounding whether patient selection, chemotherapy advancements, or radiation doses were improving outcomes.
Suker et al presented the single-arm Phase II study of SBRT (40 Gy in 5 fractions) after induction FOLFIRINOX (The LAPC-1 trial).43 They found a 1-year overall survival rate of 64% in the whole cohort (50 patients) vs 79% for patients who received SBRT after induction chemotherapy (39 patients). When these data were updated in 2021, they reported a median overall survival of 18 months for patients who received SBRT and 5 months in patients who did not.46 The reason for forgoing SBRT after induction chemotherapy was toxicity on chemotherapy (5 of 11 patients) and progression (6 of 11 patients).
Comito et al presented a Phase II study from Italy of 45 patients with LAPC who received 45 Gy in 6 fractions.47 Freedom from local progression was 90% at 2 years, and median overall survival for the cohort was 13 months after SBRT (19 months from the date of diagnosis). Thirty-two (72%) patients had received chemotherapy before enrollment; while induction therapy was not part of the trial design, these patients had not progressed while on systematic therapy because they still qualified as locally advanced at the time of enrollment.
Reyngold et al presented data from two parallel phase I studies of three fraction SBRT for LAPC.48 All 24 patients had histologically confirmed LAPC that did not progress after 2 months of induction chemotherapy. Following a 3+3 design they allocated patients to receive 3 fraction SBRT at doses of 27, 30, and 33 Gy. They did not detect any grade ≥3 toxicity or any reductions in quality-of-life scores at 3 and 6 months. The efficacy outcomes were also promising; the two-year local failure rate and overall survival were 31.7% and 29.2%, respectively.
Dose-Escalated and Adaptive SBRT
Similar to the trends seen with fractionated RT, dose–response relationships have also been demonstrated for SBRT. After the first studies of pancreatic SBRT had relatively high rates of acute and late toxicities, there was an understandable caution in pursuing dose–escalation studies.25–28 With improvements in tumor targeting through image-guidance, ablative doses can now be delivered to gross disease without compromising tolerability.49 Zhu et al performed a propensity-matched retrospective cohort study and identified that patients who received SBRT with a BED10 >70 Gy had a lower risk of solitary in-field and marginal progression compared to patients treated with BED10 between 60 and 70 Gy.50 While Zaorsky et al systematic review and meta-analysis failed to confirm this association between ablative doses of SBRT and improved local control, there are other retrospective studies demonstrating this effect.33,51 Further, a more contemporary meta-analysis by Mahadevan et al suggested a dose-response with 1-year local control of 79–86% for doses of 30–36 Gy versus 70% for doses below 24 Gy (each in 3-fraction equivalent doses).52
There has been a growing body of evidence suggesting that achieving an “ablative” biologic effective dose may be associated with comparatively favorable outcomes regardless of fractionation scheme. In a pooled analysis of patients treated with ablative doses of RT, there was no difference in outcomes in patients treated with SBRT (≤5 fractions) vs 15–25 fraction regimens.12 Given emerging evidence of the benefit of dose-escalation, one might not necessarily advocate for SBRT in patients whose anatomy precludes delivery of ablative doses.53
The proximity of PDAC to gastrointestinal luminal organs makes the safe delivery of dose-escalated RT challenging. Recent advances such as MRI-guided SBRT with adaptive planning represent one intriguing approach to overcome this challenge. Hassanzadeh et al reported a retrospective study of 44 patients treated with stereotactic magnetic resonance-guided adaptive radiation therapy (SMART) to a target dose of 50 Gy in 5 fractions.39 Gross tumor abutted a gastrointestinal luminal organ in 80% of patients, and 11% had gross tumor invasion. Despite this, they demonstrated a 1-year local control rate of 84% and favorably low grade 2 and 3 adverse event rates of 7% and 5%, respectively.
Chuong et al presented the Miami Cancer Institute experience of induction chemotherapy before SMART.40 The vast majority of patients received multiagent chemotherapy (93.6%). Then, patients received 40–50 Gy in 5 fractions (96.8% of the cohort received 50 Gy). They reported impressive 1- and 2-year local control (93.8% and 87.7%, respectively) and 1- and 2-year overall survival (90.2% and 45.5%, respectively).
Parikh et al provided the first prospective evidence of SMART (delivering 50 Gy in 5 fractions) for LAPC and BRPC.54,55 Across 13 sites, they recruited 136 patients (BRPC 59, LAPC 77) who were treated with at least 3 months of induction chemotherapy. They demonstrated tolerability (2.2% grade 3+ toxicity probably related to SBRT) and impressive 12-month and 18-month overall survival rates for the LAPC cohort (94.7% and 68.5%, respectively). These data, consistent with others’ experiences, suggest that with careful patient selection, application of induction chemotherapy, motion management, real-time image guidance, adaptive replanning, and prioritization of normal tissue sparing, escalated RT doses may be safely delivered and are associated with favorable rates of local control.13,40,56
SBRT to Bridge to Curative Intent Surgery
One proposed application of SBRT for LAPC, is the addition of SBRT to multiagent chemotherapy for patients who may be potential operative candidates. One of the first studies to prospectively evaluate this strategy was conducted by Polistina et al.38 They treated patients with neoadjuvant gemcitabine followed by SBRT with a dose of 30 Gy in 3 fractions. After SBRT patients continued gemcitabine until restaging 3 months later. They offered 5 (22%) patients exploratory surgery and possible curative intent resection; 2 patients refused, and of the remaining 3 patients, 1 had evidence of disease on peritoneal lavage. The 2 patients who underwent surgical resection successfully achieved an R0 resection and survived 17 and 20 months, respectively, whereas the median survival for the whole cohort was 10.6 months.
There are mixed estimates of resection rates for patients with LAPC from prospective and retrospective studies. For example, there were initially no resections reported in the Moffitt experience, but after it was updated in 2015, they found a resection rate of 10%.57,58 Interestingly, these investigators found no difference in survival for patients with borderline resectable pancreatic cancer and LAPC, but they did demonstrate that patients who underwent surgical resection had superior survival to those who did not. These data exemplify that while determining resectability upfront is critical to patient counseling and treatment planning, restaging and reevaluating resectability after and during neoadjuvant therapy is necessary to advance patient outcomes.
Another important trial that questions the role of SBRT for LAPC is the Alliance A021501 trial.59 The study randomized patients with borderline resectable pancreatic cancer into two parallel arms: one group received 8 cycles of FOLIFRINOX and the other received 7 cycles of FOLFIRINOX followed by SBRT. The study was designed to independently assess the 18-month overall survival of each arm compared to a historical control of 50% (ie, median survival 18 months). The study was closed early due to futility analysis, after only 10 of 30 patients in the FOLFIRINOX followed by SBRT group underwent an R0 resection. At final analysis, the 18-month overall survival was 66.7% in the FOLFIRINOX group and 47.3% in the FOLFIRINOX followed by SBRT group. Based on the pre-specified overall survival threshold, FOLFIRINOX alone was determined to represent the reference regimen for further study. While this study was conducted on borderline resectable patients, it raised the question whether SBRT should be part of the LAPC paradigm when surgery may be possible. One caveat of the study is that patients were randomized before receiving any chemotherapy instead of after completion of 7 cycles. Randomization after completing the necessary cycles of chemotherapy could have improved the balance of unmeasured confounders, which can easily bias estimates in a small study. Another limitation of the study is that SBRT was only delivered to the gross disease with a small margin, in contrast to the more contemporary preference to include a modest but more substantial elective volume encompassing patterns of spread.60 Further, in a post-hoc radiation therapy quality assurance analysis, Tchelebi et al found that 59% of cases were noted to have suboptimal contours or plan coverage.61 This study underscored the importance of rigorous quality assurance of SBRT in clinical trials and the learning curve when designing SBRT plans.
SBRT for Palliation
SBRT is a great option for patients who may not be fit for surgery due to medical comorbidities and performance status.62 Kim et al presented a series of 26 patients with ages ≥80, including a roughly equal proportion of patients with unresectable disease (54%) and resectable but poor surgical candidates (46%).63 Patients received either 24 Gy in 1 fraction or 30–36 Gy in 3 fractions. They reported reasonable local control (median 11.5 months), effective symptom relief in 8 of 10 patients who presented with abdominal pain, and no acute or late grade 3+ toxicity. This series supports the application of SBRT in elderly patients for disease control and palliation. Similarly, Moningi et al report the SBRT experience from Johns Hopkins, including 50 (57%) patients with Eastern Cooperative Oncology Group (ECOG) performance status ≥1.64 They found no significant difference in overall survival for patients with baseline or post-SBRT ECOG performance status ≥1 vs 0. While performance status might preclude a patient from receiving multiagent chemotherapy or proceeding with surgery, SBRT may be an effective option. Another series from the Medical College of Wisconsin reported the outcomes of 20 patient ages >75 treated with SBRT for LAPC.65 This cohort was particularly frail; a multidisciplinary team determined that they would not tolerate surgery or chemoradiation before proceeding with SBRT. While they reported reasonable efficacy (median overall survival of 6.4 months), 3 (15%) patients developed grade 3–4 toxicity after treatment. These cohorts and consensus statements support the application of SBRT for elderly and frail patients with pancreatic cancer who are unable to proceed to surgery.3,66
Even if patients are unable to proceed with resection after SBRT or achieve superior disease control with definitive intent SBRT, SBRT can be utilized with palliative intent to control pain. SBRT has been shown to improve pain scores in the weeks after treatment in multiple randomized trials.10 This treatment strategy is particularly effective for patients with high baseline local symptoms and for patients not receiving multiagent chemotherapy due to age, frailty, or other comorbidities.62 PDAC has a propensity for aggressive locoregional spread that involves the nearby vasculature, nerve plexi, and other organs.60 Its invasive properties can lead to neuropathic, visceral, and somatic pain.67 A systematic review of SBRT for controlling pain was presented by Buwenge et al in 2018.10 Their review had several limitations including the high level of heterogeneity and the lack of consistent standards for reporting pain relief in studies of LAPC, however they found an 85% overall response rate (complete and partial responses) of pain after SBRT. Further, beyond the conventional approach of treating the primary pancreatic mass to control pain symptoms, investigators from Israel have been exploring the role of celiac ablation in patients with PDAC.68 With just a single treatment (25 Gy in 1 fraction) delivered partially to the tumor but primarily to the anterolateral aorta from the twelfth thoracic to second lumbar vertebra, they achieved meaningful pain relief (a decrease of ≥2 points on the Numerical Rating Scale) in 76% of evaluable patients at 3 weeks. There was some loss to follow up their response rate, which increased to 92% at 6 weeks. This approach was safe with no grade 3 toxicities reported, allowing for a convenient and safe single-visit approach for improving PDAC-related pain.
Planning and Treatment Considerations
An example of the workflow for diagnosis and management of LAPC is shown in Figure 1. Patients diagnosed with PDAC should routinely undergo multidisciplinary evaluation to determine the most appropriate treatment.69 If a patient is diagnosed with LAPC, multiagent chemotherapy (eg, FOLFIRINOX or gemcitabine/nab-paclitaxel) is most frequently offered as a frontline treatment.3 After several cycles of chemotherapy, patients are restaged with CT imaging and if their disease has responded or is stable, a (re)consultation with a radiation oncologist is recommended to consider local therapy.
![]() |
Figure 1 Diagnosis and Management Workflow for Patients with LAPC. |
In patients planned for definitive RT, a CT and/or MRI simulation (preferably with intravenous contrast) should be performed. Placement of peri-tumoral fiducial markers prior to simulation to assist with daily image guidance may be considered because bony anatomy and biliary stents may be relatively poor surrogates for the gross pancreas tumor.70,71 Of note, fiducials are not needed with MR-guided SBRT because of the superior soft-tissue resolution with MRI. Patients are instructed to fast at least 2–3 hours prior to CT simulation and treatment to restrict stomach/duodenum filling to maintain reproducible anatomy and because it often retracts the stomach away from the target volume, thereby reducing radiation exposure. Patient-specific respiratory motion assessment and management should be determined at the time of simulation. Employing techniques such as breath-hold, phase-based gating, and abdominal compression are critical in restricting the magnitude of tumor motion throughout the respiratory cycle.53,72,73 Intra-fraction motion assessment can also be utilized to enhance the precision of treatment delivery.74 MRI and CT-based online adaptive radiation platforms also enable the possibility of daily adjustments of treatment plans to account for variability in the position of the stomach and small bowel during each fraction.
In terms of treatment planning, the proximity of gross tumor to GI luminal organs (eg, stomach, small bowel, and duodenum) is a major concern as the GI luminal organs are highly sensitive to RT and increasingly susceptible to injury with higher dose per fraction. Limiting high-dose RT exposure to such organs remains highest priority during RT plan optimization. When attempting dose-escalation when gross tumor is in relatively close proximity to bowel, 2 predominant practices have emerged: 1) maintaining a 5-fraction SBRT regimen targeting escalated RT doses to the majority of gross tumor while accepting under-coverage adjacent to GI luminal organs or 2) delivering a more protracted high BED RT course over 15–25 fractions, at a lower dose per fraction, which may be more forgiving as it relates to risk of injury to GI luminal organs. Each of these approaches uses a “dose-painting” strategy in which a heterogeneous radiation-dose distribution is developed to allow maximal coverage of target while limiting GI luminal organ exposure within previously established radiation-dose thresholds associated with toxicity. Such an approach inherently limits target coverage of regions adjacent to bowel to more “conventional” radiation doses. This is generally preferred when weighing the risks and benefits associated with marginal improvements in local control vs heightened risk of severe RT-associated adverse effects. Similarly, in potentially operable patients with LAPC, a “dose-painting” strategy can also be utilized to deliver escalated RT doses to regions at high-risk of compromised margins, such as the tumor–vessel interface.60 Example RT treatment plans are shown in Figure 2.
Regarding RT target volumes, both the ASTRO and ESTRO/ACROP guidelines suggest targeting the gross tumor volume through all stages of the respiratory cycle (iGTV) with a small volume expansion of 0.2–0.5 cm to account for variation in daily setup.4,75 Recognizing the infiltrative nature of PDAC with high rates of occult perineural invasion (~90%) and regional lymph node involvement (>60–70%) identified in surgical series for patients with anatomically resectable disease, along with conventional imaging’s underestimation of gross tumor size, many clinicians advocate for a lower RT dose elective target volume including expansions of approximately 0.5–1.5 cm upon the primary tumor and elective regional lymph node regions.60 Retrospective data do suggest improved locoregional control and pathologic surrogate outcomes (eg, pathologic lymph node involvement) amongst operable patients with such approaches; however, no OS benefit has been demonstrated.76–79 It is also recognized that with less conformal RT strategies (eg, 3D conformal RT), incidental radiation exposure to these at risk-regions can approach 70–80% of prescription dose even when not explicitly targeted.80 However, in the context of highly conformal SBRT, deliberately targeting elective nodes should be considered as incidental exposure will be considerably less than with conventional RT techniques.81
Future Directions
SBRT for LAPC has continued to evolve. One exciting area of development is using blood- and imaging-based biomarkers for selecting patients for treatment intensification with SBRT. A study from MD Anderson Cancer Center assessed predictors of a pathologic major response (pMR; 82 They found that low post-treatment carbohydrate antigen 19–9 (CA 19–9) levels, partial response by Response Evaluation Criteria in Solid Tumors (RECIST) 1.1, and reduction of tumor volume were associated with pMR. Integration of these metrics for the LAPC population after induction chemotherapy may help stratify patients who need treatment intensification due to residual disease or who may have the best chance to ultimately proceed to resection. A study by Lee et al evaluated the association between FDG-PET-based parameters and clinical outcomes in patients with BRPC and LAPC.83 They found that dynamics in PET-based metrics and post-neoadjuvant chemotherapy measurements of metabolic activity are predictive of recurrence and overall survival. Similar to changes in restaging CT scan and CA 19–9 kinetics, PET-CT or PET-MRI can improve prognostication and stratification prior to SBRT. There is also a growing interest in the use of histological and blood-based biomarkers for stratification of patients with LAPC beyond measuring CA 19–9. One example was born out of an autopsy series from Johns Hopkins University; Iacobuzio-Donahue et al analyzed histological 76 patients with pancreatic cancer.84 They found that only 22% of patients with locally advanced PDAC without metastatic disease at autopsy had loss of expression of Dpc4/SMAD4. They also demonstrated that Dpc4/SMAD4 loss was found in 78% of patients who had hundreds to thousands of metastases. When analyzing the patterns of failure, they found that a metastatic phenotype, as opposed to a locally destructive phenotype, was more commonly associated with Dpc4/SMAD4 loss. These findings led investigators to consider the possible utility of measuring Dpc4/SMAD4 expression when selecting patients for locoregional treatment, hypothesizing that patients with intact Dpc4/SMAD4 expression might benefit most from RT.85 More recently, another study from Johns Hopkins evaluated the utility of measuring circulating tumor cells in peripheral blood of patients with PDAC. They found that detection of CTCs expressing vimentin and cytokeratin was associated with recurrence. One could envision future trials stratifying patients who may be at higher risk of recurrence for chemotherapy only approaches as they would likely not benefit from superior locoregional control.
Beyond advancements in the delivery of treatment with adaptive technologies, investigators have also tested combination treatments to widen the therapeutic window. One example is Rucosopasem Manganese (GC4711), an investigational superoxide dismutase mimetic that converts superoxide to hydrogen peroxide. GC4711 is a second-generation superoxide dismutase mimetic that is thought to improve upon the radioprotective qualities of Avasopasem manganese (GC4419) that demonstrated favorable results in a phase Ib/II trial.86 GC4711is hypothesized to increase the tolerability and efficacy of SBRT as normal cells can metabolize hydrogen peroxide better than cancer cells. In a phase I/IIa trial of patients with LAPC, there were no signs of unanticipated early or late toxicity from the combination of SBRT and GC4711.87 Interestingly, while there was only a non-significant increase in locoregional control with GC4711 (HR = 0.30, p = 0.06), there was a significant prolongation of distant metastases control (disease outside of RT field) with GC4711 (HR = 0.39, p = 0.03). In this small study, there was a non-significant improvement in survival with GC4711 (HR = 0.48, p = 0.09), and many were waiting in anticipation for the maturation of the results of the confirmatory Phase IIb trial, GRECO-2.88–90 Unfortunately, the GRECO-2 study was halted early after a futility analysis of the first 177 patients.91 That being said, there remains enthusiasm for adding therapeutic agents, such as gadolinium-based nanoparticles, to improve the tolerability and efficacy of dose-escalated SBRT (NCT04789486).
While the GRECO-2 study was designed to test the utility of adding GC4711, the Locally Advanced Pancreatic Cancer Treated With ABLAtivE Stereotactic MRI-guided Adaptive Radiation Therapy (LAP-ABLATE) trial was designed to definitively test if there is any benefit in adding SMART to induction multiagent chemotherapy (NCT05585554). The study planned to test the role of applying adaptive technology when delivering ablative doses of SBRT vs treatment with chemotherapy alone. With a planned enrollment of 267 participants, the study was one of the largest of LAPC ever initiated. Unfortunately, the trial was terminated due to industry sponsor financial concerns. Prospective evaluation of the benefit of adding dose-escalated SBRT to multiagent chemotherapy remains an important question.
Another trial that may inform clinical practice is the randomized phase II study of FOLFIRINOX and Stereotactic Radiotherapy (SBRT) for Pancreatic Cancer With High Risk and Locally Advanced Disease (MASTERPLAN).92 The study is recruiting 120 patients, randomized between systemic therapy alone vs systemic therapy followed by SBRT (40 Gy in 5 fractions) delivered over two weeks. While the SBRT dose delivered has 25% lower biologically effective dose than the 50 Gy regimens used in other trials, the doses used are still technically ablative by some standards (BED10 > 70 Gy).
Beyond testing whether SBRT provides superior efficacy over systemic therapy alone, there are additional studies investigating the optimal systemic therapy when combined with SBRT. The sequential treatment with GEMBRAX and then FOLFIRINOX followed by stereotactic MRI-guided radiotherapy in patients with locally advanced pancreatic cancer (GABRINOX-ART) trial will evaluate if sequential treatment with two highly effective systemic therapy regimens prior to SMART will be feasible (NCT04570943).
While there is a growing body of evidence supporting the role of dose-escalated SBRT for LAPC, there has not been a randomized study proving benefit. Investigators from Changhai Hospital have proposed a randomized study comparing SBRT with a biologically effective dose of 60–70 Gy vs ablative doses (BED > 70 Gy) (NCT04603586).93
Conclusion
There is a growing body of evidence supporting the safety and efficacy of SBRT for LAPC. The field has evolved considerably from the early 2000s; from single fraction SBRT to the utilization of MRI guidance and adaptation, SBRT has come a long way. Advances in SBRT delivery have allowed for the safe delivery of ablative doses, which provides an opportunity for advancing outcomes for this challenging to treat population. In practice, SBRT has a critical role in the definitive and palliative treatment of patients with advanced pancreatic cancer. Even so, there is still a paucity of randomized evidence supporting an overall survival benefit of SBRT. We wait anxiously for the maturation of several Phase 3 studies clarifying the role of SBRT for LAPC.
Disclosure
Dr Krishan Jethwa reports personal fees from RadoncQuestions.com, LLC, during the conduct of the study. The authors report no other conflicts of interest in this work.
References
1. Siegel RL, Miller KD, Fuchs HE, Jemal A. Cancer statistics, 2022. CA. 2022;72(1):7–33. doi:10.3322/caac.21708
2. Vincent A, Herman J, Schulick R, Hruban RH, Goggins M. Pancreatic cancer. Lancet. 2011;378(9791):607–620. doi:10.1016/s0140-6736(10)62307-0
3. Balaban EP, Mangu PB, Khorana AA, et al. Locally advanced, unresectable pancreatic cancer: American society of clinical oncology clinical practice guideline. J Clin Oncol. 2016;34(22):2654–2668. doi:10.1200/jco.2016.67.5561
4. Palta M, Godfrey D, Goodman KA, et al. Radiation therapy for pancreatic cancer: executive summary of an ASTRO clinical practice guideline. Pract Radiat Oncol. 2019;9(5):322–332. doi:10.1016/j.prro.2019.06.016
5. Hammel P, Huguet F, van Laethem J-L, et al. Effect of chemoradiotherapy vs chemotherapy on survival in patients with locally advanced pancreatic cancer controlled after 4 months of gemcitabine with or without erlotinib: the LAP07 randomized clinical trial. JAMA. 2016;315(17):1844–1853.
6. Klaassen DJ, Macintyre JM, Catton GE, Engstrom PF, Moertel CG. Treatment of locally unresectable cancer of the stomach and pancreas: a randomized comparison of 5-fluorouracil alone with radiation plus concurrent and maintenance 5-fluorouracil–an eastern cooperative oncology group study. J Clin Oncol. 1985;3(3):373–378. doi:10.1200/jco.1985.3.3.373
7. Moertel CG, Frytak S, Hahn RG, et al. Therapy of locally unresectable pancreatic carcinoma: a randomized comparison of high dose (6000 rads) radiation alone, moderate dose radiation (4000 rads + 5-fluorouracil), and high dose radiation + 5-fluorouracil: the gastrointestinal tumor study group. Cancer. 1981;48(8):1705–1710. doi:10.1002/1097-0142(19811015)48:83.0.co;2-4
8. Gastrointestinal Tumor Study Group. Treatment of locally unresectable carcinoma of the pancreas: comparison of combined-modality therapy (chemotherapy plus radiotherapy) to chemotherapy alone. JNCI J National Cancer Inst. 1988;80(10):751–755. doi:10.1093/jnci/80.10.751
9. Gemenetzis G, Groot VP, Blair AB, et al. Survival in locally advanced pancreatic cancer after neoadjuvant therapy and surgical resection. Ann Surg. 2019;270(2):340–347. doi:10.1097/sla.0000000000002753
10. Buwenge M, Macchia G, Arcelli A, et al. Stereotactic radiotherapy of pancreatic cancer: a systematic review on pain relief. J Pain Res. 2018;11:2169–2178. doi:10.2147/jpr.s167994
11. Reyngold M, O’Reilly EM, Varghese AM, et al. Association of ablative radiation therapy with survival among patients with inoperable pancreatic cancer. JAMA Oncol. 2021;7(5):735–738. doi:10.1001/jamaoncol.2021.0057
12. Reyngold M, O’Reilly E, Herrera R, et al. Multi-institutional comparison of ablative radiation therapy in 5 versus 15–25 fractions for locally advanced pancreatic cancer. Int J Radiat Oncol Biol Phys. 2022;114(3):S106. doi:10.1016/j.ijrobp.2022.07.534
13. Chuong MD, Kirsch C, Herrera R, et al. Long-term multi-institutional outcomes of 5-fraction ablative stereotactic mr-guided adaptive radiation therapy (SMART) for inoperable pancreas cancer with median prescribed biologically effective dose of 100 Gy10. Int J Radiat Oncol Biol Phys. 2021;111(3):S147–S148. doi:10.1016/j.ijrobp.2021.07.330
14. Timmerman RD, Kavanagh BD, Cho LC, Papiez L, Xing L. Stereotactic body radiation therapy in multiple organ sites. J Clin Oncol. 2007;25(8):947–952.
15. Shinchi H, Takao S, Noma H, et al. Length and quality of survival after external-beam radiotherapy with concurrent continuous 5-fluorouracil infusion for locally unresectable pancreatic cancer. Int J Radiat Oncol Biol Phys. 2002;53(1):146–150. doi:10.1016/s0360-3016(01)02806-1
16. Loehrer PJ, Feng Y, Cardenes H, et al. Gemcitabine alone versus gemcitabine plus radiotherapy in patients with locally advanced pancreatic cancer: an eastern cooperative oncology group trial. J Clin Oncol. 2011;29(31):4105–4112. doi:10.1200/jco.2011.34.8904
17. Huguet F, André T, Hammel P, et al. Impact of chemoradiotherapy after disease control with chemotherapy in locally advanced pancreatic adenocarcinoma in GERCOR phase ii and iii studies. J Clin Oncol. 2007;25(3):326–331. doi:10.1200/jco.2006.07.5663
18. Huguet F, Girard N, Guerche CS-E, Hennequin C, Mornex F, Azria D. Chemoradiotherapy in the management of locally advanced pancreatic carcinoma: a qualitative systematic review. J Clin Oncol. 2009;27(13):2269–2277. doi:10.1200/jco.2008.19.7921
19. Conroy T, Desseigne F, Ychou M, et al. FOLFIRINOX versus gemcitabine for metastatic pancreatic cancer. N Engl J Med. 2011;364(19):1817–1825. doi:10.1056/nejmoa1011923
20. Von Hoff DD, Ervin T, Arena FP, et al. Increased survival in pancreatic cancer with nab-paclitaxel plus gemcitabine. N Engl J Med. 2013;369(18):1691–1703. doi:10.1056/nejmoa1304369
21. Philip PA, Lacy J, Portales F, et al. Nab-paclitaxel plus gemcitabine in patients with locally advanced pancreatic cancer (LAPACT): a multicentre, open-label Phase 2 study. Lancet Gastroenterol Hepatol. 2020;5(3):285–294. doi:10.1016/s2468-1253(19)30327-9
22. Ozaka M, Ueno M, Ishii H, et al. Randomized phase II study of modified FOLFIRINOX versus gemcitabine plus nab-paclitaxel combination therapy for locally advanced pancreatic cancer (JCOG1407). J Clin Oncol. 2021;39(15_suppl):4017. doi:10.1200/JCO.2021.39.15_suppl.4017
23. Ducreux M, Desgrippes R, Rinaldi Y, et al. 1296MO PRODIGE 29-UCGI 26 (NEOPAN): a Phase III randomised trial comparing chemotherapy with folfirinox or gemcitabine in locally advanced pancreatic carcinoma (LAPC). Ann Oncol. 2022;33:S1136.
24. Fietkau R, Ghadimi M, Grützmann R, et al. Randomized phase III trial of induction chemotherapy followed by chemoradiotherapy or chemotherapy alone for nonresectable locally advanced pancreatic cancer: first results of the CONKO-007 trial. J Clin Oncol. 2022;40(16_suppl):4008. doi:10.1200/jco.2022.40.16_suppl.4008
25. Koong AC, Le QT, Ho A, et al. Phase I study of stereotactic radiosurgery in patients with locally advanced pancreatic cancer. Int J Radiat Oncol Biol Phys. 2004;58(4):1017–1021. doi:10.1016/j.ijrobp.2003.11.004
26. Schellenberg D, Goodman KA, Lee F, et al. Gemcitabine chemotherapy and single-fraction stereotactic body radiotherapy for locally advanced pancreatic cancer. Int J Radiat Oncol Biol Phys. 2008;72(3):678–686. doi:10.1016/j.ijrobp.2008.01.051
27. Koong AC, Christofferson E, Le Q-T, et al. Phase II study to assess the efficacy of conventionally fractionated radiotherapy followed by a stereotactic radiosurgery boost in patients with locally advanced pancreatic cancer. Int J Radiat Oncol Biol Phys. 2005;63(2):320–323. doi:10.1016/j.ijrobp.2005.07.002
28. Chang DT, Schellenberg D, Shen J, et al. Stereotactic radiotherapy for unresectable adenocarcinoma of the pancreas. Cancer. 2009;115(3):665–672. doi:10.1002/cncr.24059
29. Hoyer M, Roed H, Sengelov L, et al. Phase-II study on stereotactic radiotherapy of locally advanced pancreatic carcinoma. Radiother Oncol. 2005;76(1):48–53. doi:10.1016/j.radonc.2004.12.022
30. Schellenberg D, Kim J, Christman-Skieller C, et al. Single-fraction stereotactic body radiation therapy and sequential gemcitabine for the treatment of locally advanced pancreatic cancer. Int J Radiat Oncol Biol Phys. 2011;81(1):181–188. doi:10.1016/j.ijrobp.2010.05.006
31. Mahadevan A, Jain S, Goldstein M, et al. Stereotactic body radiotherapy and gemcitabine for locally advanced pancreatic cancer. Int J Radiat Oncol Biol Phys. 2010;78(3):735–742. doi:10.1016/j.ijrobp.2009.08.046
32. Mahadevan A, Miksad R, Goldstein M, et al. Induction gemcitabine and stereotactic body radiotherapy for locally advanced nonmetastatic pancreas cancer. Int J Radiat Oncol Biol Phys. 2011;81(4):e615–22. doi:10.1016/j.ijrobp.2011.04.045
33. Petrelli F, Comito T, Ghidini A, Torri V, Scorsetti M, Barni S. Stereotactic body radiation therapy for locally advanced pancreatic cancer: a systematic review and pooled analysis of 19 trials. Int J Radiat Oncol Biol Phys. 2017;97(2):313–322. doi:10.1016/j.ijrobp.2016.10.030
34. Park JJ, Hajj C, Reyngold M, et al. Stereotactic body radiation vs. intensity-modulated radiation for unresectable pancreatic cancer. Acta Oncologica. 2017;56(12):1746–1753. doi:10.1080/0284186x.2017.1342863
35. Abi Jaoude J, Thunshelle CP, Kouzy R, et al. Stereotactic versus conventional radiation therapy for patients with pancreatic cancer in the modern era. Adv Radiat Oncol. 2021;6(6):100763. doi:10.1016/j.adro.2021.100763
36. Arcelli A, Buwenge M, Macchia G, et al. Stereotactic body radiotherapy vs conventionally fractionated chemoradiation in locally advanced pancreatic cancer: a multicenter case‐control study (PAULA‐1). Cancer Med. 2020;9(21):7879–7887. doi:10.1002/cam4.3330
37. Tchelebi LT, Lehrer EJ, Trifiletti DM, et al. Conventionally fractionated radiation therapy versus stereotactic body radiation therapy for locally advanced pancreatic cancer (CRiSP): an international systematic review and meta‐analysis. Cancer. 2020;126(10):2120–2131. doi:10.1002/cncr.32756
38. Polistina F, Costantin G, Casamassima F, et al. Unresectable locally advanced pancreatic cancer: a multimodal treatment using neoadjuvant chemoradiotherapy (gemcitabine plus stereotactic radiosurgery) and subsequent surgical exploration. Ann Surg Oncol. 2010;17(8):2092–2101. doi:10.1245/s10434-010-1019-y
39. Hassanzadeh C, Rudra S, Bommireddy A, et al. Ablative five-fraction stereotactic body radiation therapy for inoperable pancreatic cancer using online MR-guided adaptation. Adv Radiat Oncol. 2021;6(1):100506. doi:10.1016/j.adro.2020.06.010
40. Chuong MD, Herrera R, Kaiser A, et al. Induction chemotherapy and ablative stereotactic magnetic resonance image-guided adaptive radiation therapy for inoperable pancreas cancer. Front Oncol. 2022;12:888462. doi:10.3389/fonc.2022.888462
41. Herman JM, Chang DT, Goodman KA, et al. Phase 2 multi‐institutional trial evaluating gemcitabine and stereotactic body radiotherapy for patients with locally advanced unresectable pancreatic adenocarcinoma. Cancer. 2015;121(7):1128–1137. doi:10.1002/cncr.29161
42. Liauw SL, Ni L, Wu T, et al. A prospective trial of stereotactic body radiation therapy for unresectable pancreatic cancer testing ablative doses. J Gastrointest Oncol. 2020;11(6):1399–1407. doi:10.21037/jgo-20-187
43. Suker M, Nuyttens JJ, Eskens FALM, et al. Efficacy and feasibility of stereotactic radiotherapy after folfirinox in patients with locally advanced pancreatic cancer (LAPC-1 trial). EClinicalMedicine. 2019;17:100200. doi:10.1016/j.eclinm.2019.10.013
44. Suker M, Beumer BR, Sadot E, et al. FOLFIRINOX for locally advanced pancreatic cancer: a systematic review and patient-level meta-analysis. Lancet Oncol. 2016;17(6):801–810. doi:10.1016/s1470-2045(16)00172-8
45. Quan K, Sutera P, Xu K, et al. Results of a prospective phase 2 clinical trial of induction gemcitabine/capecitabine followed by stereotactic ablative radiation therapy in borderline resectable or locally advanced pancreatic adenocarcinoma. Pract Radiat Oncol. 2018;8(2):95–106. doi:10.1016/j.prro.2017.10.001
46. Teriaca MA, Loi M, Suker M, Eskens F, van Eijck CHJ, Nuyttens JJ. A phase II study of stereotactic radiotherapy after FOLFIRINOX for locally advanced pancreatic cancer (LAPC-1 trial): long-term outcome. Radiother Oncol. 2021;155:232–236. doi:10.1016/j.radonc.2020.11.006
47. Comito T, Cozzi L, Clerici E, et al. Can stereotactic body radiation therapy be a viable and efficient therapeutic option for unresectable locally advanced pancreatic adenocarcinoma? Results of a phase 2 study. Technol Cancer Res Treat. 2017;16(3):295–301. doi:10.1177/1533034616650778
48. Reyngold M, Karam SD, Hajj C, et al. Phase 1 dose escalation study of SBRT using 3 fractions for locally advanced pancreatic cancer. Int J Radiat Oncol Biol Phys. 2023. doi:10.1016/j.ijrobp.2023.03.036
49. Zaorsky NG, Lehrer EJ, Handorf E, Meyer JE. Dose escalation in stereotactic body radiation therapy for pancreatic cancer: a meta-analysis. Am J Clin Oncol. 2019;42(1):46–55. doi:10.1097/coc.0000000000000472
50. Zhu X, Cao Y, Su T, et al. Failure patterns and outcomes of dose escalation of stereotactic body radiotherapy for locally advanced pancreatic cancer: a multicenter cohort study. Therapeut Adv Med Oncol. 2020;12:175883592097715. doi:10.1177/1758835920977155
51. Zhu X, Ju X, Cao Y, et al. Patterns of local failure after stereotactic body radiation therapy and sequential chemotherapy as initial treatment for pancreatic cancer: implications of target volume design. Int J Radiat Oncol Biol Phys. 2019;104(1):101–110. doi:10.1016/j.ijrobp.2019.01.075
52. Mahadevan A, Moningi S, Grimm J, et al. Maximizing tumor control and limiting complications with stereotactic body radiation therapy for pancreatic cancer. Int J Radiat Oncol Biol Phys. 2021;110(1):206–216. doi:10.1016/j.ijrobp.2020.11.017
53. Reyngold M, Parikh P, Crane CH. Ablative radiation therapy for locally advanced pancreatic cancer: techniques and results. Radiat Oncol. 2019;14(1):1–8.
54. Parikh P, Lee P, Low D, et al. Stereotactic MR-guided on-table adaptive radiation therapy (SMART) for patients with borderline or locally advanced pancreatic cancer: primary endpoint outcomes of a prospective phase II multi-center international trial. Int J Radiat Oncol Biol Phys. 2022;114(5):1062–1063.
55. Parikh PJ, Lee P, Low DA, et al. A multi-institutional phase II trial of ablative 5-fraction stereotactic MR-guided on-table adaptive radiation therapy for borderline resectable and locally advanced pancreatic cancer. Int J Radiat Oncol Biol Phys. 2023. doi:10.1016/j.ijrobp.2023.05.023
56. Rossi G, Simoni N, Paiella S, et al. Risk adapted ablative radiotherapy after intensive chemotherapy for locally advanced pancreatic cancer. Front Oncol. 2021;11. doi:10.3389/fonc.2021.662205
57. Mellon EA, Hoffe SE, Springett GM, et al. Long-term outcomes of induction chemotherapy and neoadjuvant stereotactic body radiotherapy for borderline resectable and locally advanced pancreatic adenocarcinoma. Acta Oncologica. 2015;54(7):979–985. doi:10.3109/0284186x.2015.1004367
58. Chuong MD, Springett GM, Freilich JM, et al. Stereotactic body radiation therapy for locally advanced and borderline resectable pancreatic cancer is effective and well tolerated. Int J Radiat Oncol Biol Phys. 2013;86(3):516–522. doi:10.1016/j.ijrobp.2013.02.022
59. Katz MHG, Shi Q, Meyers J, et al. Efficacy of preoperative mFOLFIRINOX vs mFOLFIRINOX plus hypofractionated radiotherapy for borderline resectable adenocarcinoma of the pancreas: the A021501 phase 2 randomized clinical trial. JAMA Oncol. 2022;8(9):1263–1270. doi:10.1001/jamaoncol.2022.2319
60. Jethwa KR, Neibart SS, Truty MJ, Jabbour SK, Hallemeier CL. Patterns of recurrence after primary local therapy for pancreatic ductal adenocarcinoma – A critical review of rationale and target delineation for (Neo)adjuvant radiation therapy. Pract Radiat Oncol. 2022;12(6):e463–e473. doi:10.1016/j.prro.2022.06.004
61. Tchelebi LT, Segovia D, Smith K, et al. Radiation therapy quality assurance analysis of alliance A021501: preoperative mFOLFIRINOX or mFOLFIRINOX plus hypofractionated radiation therapy for borderline resectable adenocarcinoma of the pancreas. Int J Radiat Oncol Biol Phys. 2024. doi:10.1016/j.ijrobp.2024.03.013
62. Rosati LM, Herman JM. Role of stereotactic body radiotherapy in the treatment of elderly and poor performance status patients with pancreatic cancer. J Oncol Pract. 2017;13(3):157–166. doi:10.1200/jop.2016.020628
63. Kim CH, Ling DC, Wegner RE, et al. Stereotactic body radiotherapy in the treatment of Pancreatic Adenocarcinoma in elderly patients. Radiat Oncol. 2013;8(1):240. doi:10.1186/1748-717x-8-240
64. Moningi S, Dholakia AS, Raman SP, et al. The role of stereotactic body radiation therapy for pancreatic cancer: a single-institution experience. Ann Surg Oncol. 2015;22(7):2352–2358. doi:10.1245/s10434-014-4274-5
65. Yechieli RL, Robbins JR, Mahan M, Siddiqui F, Ajlouni M. Stereotactic body radiotherapy for elderly patients with medically inoperable pancreatic cancer. Am J Clin Oncol. 2017;40(1):22–26. doi:10.1097/coc.0000000000000090
66. Kunkler IH, Audisio R, Belkacemi Y, et al. Review of current best practice and priorities for research in radiation oncology for elderly patients with cancer: the international society of geriatric oncology (SIOG) task force. Ann Oncol. 2014;25(11):2134–2146. doi:10.1093/annonc/mdu104
67. Lohse I, Brothers SP. Pathogenesis and treatment of pancreatic cancer related pain. Anticancer Res. 2020;40(4):1789–1796. doi:10.21873/anticanres.14133
68. Hammer L, Hausner D, Ben-Ayun M, et al. Single-fraction celiac plexus radiosurgery: a preliminary proof-of-concept phase 2 clinical trial. Int J Radiat Oncol Biol Phys. 2022;113(3):588–593. doi:10.1016/j.ijrobp.2022.02.038
69. Pawlik TM, Laheru D, Hruban RH, et al. Evaluating the impact of a single-day multidisciplinary clinic on the management of pancreatic cancer. Ann Surg Oncol. 2008;15(8):2081–2088. doi:10.1245/s10434-008-9929-7
70. Pepin E, Olsen L, Badiyan S, et al. Comparison of implanted fiducial markers and self-expandable metallic stents for pancreatic image guided radiation therapy localization. Pract Radiat Oncol. 2015;5(3):e193–e199. doi:10.1016/j.prro.2014.08.019
71. van der Horst A, Lens E, Wognum S, et al. Limited role for biliary stent as surrogate fiducial marker in pancreatic cancer: stent and intratumoral fiducials compared. Int J Radiat Oncol Biol Phys. 2014;89(3):641–648. doi:10.1016/j.ijrobp.2014.03.029
72. Tyagi N, Liang J, Burleson S, et al. Feasibility of ablative stereotactic body radiation therapy of pancreas cancer patients on a 1.5 Tesla magnetic resonance-linac system using abdominal compression. Phys Imaging Radiat Oncol. 2021;19:53–59. doi:10.1016/j.phro.2021.07.006
73. Campbell WG, Jones BL, Schefter T, Goodman KA, Miften M. An evaluation of motion mitigation techniques for pancreatic SBRT. Radiother Oncol. 2017;124(1):168–173. doi:10.1016/j.radonc.2017.05.013
74. Zeng C, Li X, Lu W, et al. Accuracy and efficiency of respiratory gating comparable to deep inspiration breath hold for pancreatic cancer treatment. J Appl Clin Med Phys. 2021;22(1):218–225. doi:10.1002/acm2.13137
75. Brunner TB, Haustermans K, Huguet F, et al. ESTRO ACROP guidelines for target volume definition in pancreatic cancer. Radiother Oncol. 2021;154:60–69. doi:10.1016/j.radonc.2020.07.052
76. Miller JA, Toesca DAS, Baclay JRM, et al. Pancreatic stereotactic body radiation therapy with or without hypofractionated elective nodal irradiation. Int J Radiat Oncol Biol Phys. 2022;112(1):131–142. doi:10.1016/j.ijrobp.2021.07.1698
77. Barrord M, Ahmad S, Patel S, et al. Patterns of failure after neoadjuvant stereotactic body radiation therapy or fractionated chemoradiation in resectable and borderline resectable pancreatic cancer. Pancreas. 2020;49(7):941–946. doi:10.1097/mpa.0000000000001602
78. Hill C, Sehgal S, Fu W, et al. High local failure rates despite high margin-negative resection rates in a cohort of borderline resectable and locally advanced pancreatic cancer patients treated with stereotactic body radiation therapy following multi-agent chemotherapy. Cancer Med. 2022;11(7):1659–1668. doi:10.1002/cam4.4527
79. Mokhtech M, Miccio JA, Johung K, et al. Multiagent chemotherapy followed by stereotactic body radiotherapy versus conventional radiotherapy for resected pancreas cancer. Am J Clin Oncol. 2022;45(11):450–457. doi:10.1097/coc.0000000000000947
80. Fokas E, Eccles C, Patel N, et al. A treatment planning comparison of four target volume contouring guidelines for locally advanced pancreatic cancer radiotherapy. Radiother Oncol. 2013;107(2):200–206. doi:10.1016/j.radonc.2013.04.010
81. Kharofa J, Mierzwa M, Olowokure O, et al. Pattern of marginal local failure in a phase ii trial of neoadjuvant chemotherapy and stereotactic body radiation therapy for resectable and borderline resectable pancreas cancer. Am J Clin Oncol. 2019;42(3):247–252. doi:10.1097/coc.0000000000000518
82. Perri G, Prakash L, Wang H, et al. Radiographic and serologic predictors of pathologic major response to preoperative therapy for pancreatic cancer. Ann Surg. 2021;273(4):806–813. doi:10.1097/sla.0000000000003442
83. Lee W, Oh M, Kim JS, et al. Metabolic activity by FDG-PET/CT after neoadjuvant chemotherapy in borderline resectable and locally advanced pancreatic cancer and association with survival. Br J Surg. 2021;109(1):61–70. doi:10.1093/bjs/znab229
84. Iacobuzio-Donahue CA, Fu B, Yachida S, et al. DPC4 gene status of the primary carcinoma correlates with patterns of failure in patients with pancreatic cancer. J Clin Oncol. 2009;27(11):1806. doi:10.1200/JCO.2008.17.7188
85. Crane CH, Varadhachary GR, Yordy JS, et al. Phase II trial of cetuximab, gemcitabine, and oxaliplatin followed by chemoradiation with cetuximab for locally advanced (T4) pancreatic adenocarcinoma: correlation of Smad4(Dpc4) immunostaining with pattern of disease progression. J Clin Oncol. 2011;29(22):3037–3043. doi:10.1200/jco.2010.33.8038
86. Taniguchi CM, Frakes JM, Aguilera TA, et al. Stereotactic body radiotherapy with or without selective dismutase mimetic in pancreatic adenocarcinoma: an adaptive, randomised, double-blind, placebo-controlled, phase 1b/2 trial. Lancet Oncol. 2023;24(12):1387–1398. doi:10.1016/s1470-2045(23)00478-3
87. Galera therapeutics corporate deck. Galera Therapeutics; 2022. Available from: https://investors.galeratx.com/events-and-presentations.
88. Hoffe S, Kim DW, Malafa M, et al. GRECO-2: a randomized, phase 2 study of stereotactic body radiation therapy (SBRT) in combination with GC4711 in the treatment of unresectable or borderline resectable nonmetastatic pancreatic cancer (PC). Int J Radiat Oncol Biol Phys. 2021;111(3):e44–e45. doi:10.1016/j.ijrobp.2021.07.372
89. Hoffe SE, Kim DW, Costello J, et al. GRECO-2: a randomized, phase 2 study of stereotactic body radiation therapy (SBRT) in combination with GC4711 in the treatment of unresectable or borderline resectable nonmetastatic pancreatic cancer (PC). J Clin Oncol. 2021;39(15_suppl):TPS4175–TPS4175. doi:10.1200/JCO.2021.39.15_suppl.TPS4175
90. Hoffe SE, Kim DW, Costello J, et al. GRECO-2: a randomized, phase 2 study of stereotactic body radiation therapy (SBRT) in combination with rucosopasem (GC4711) in the treatment of locally advanced or borderline resectable nonmetastatic pancreatic cancer. J Clin Oncol. 2022;40(16_suppl):TPS4184–TPS4184. doi:10.1200/jco.2022.40.16_suppl.tps4184
91. Therapeutics G Galera announces receipt of type A meeting minutes and strategic update; 2023.
92. Oar A, Lee M, Le H, et al. AGITG MASTERPLAN: a randomised phase II study of modified FOLFIRINOX alone or in combination with stereotactic body radiotherapy for patients with high-risk and locally advanced pancreatic cancer. BMC Cancer. 2021;21(1):1.
93. Ye Y, Zhu X, Zhao X, Jiang L, Cao Y, Zhang H. Biologically effective doses of 60–70Gy versus >70Gy of stereotactic body radiotherapy (SBRT) combined with chemotherapy in locally advanced pancreatic cancer: protocol of a single-centre, phase II clinical trial. BMJ Open. 2022;12(8):e049382. doi:10.1136/bmjopen-2021-049382