Fitness
TAK1 alleviates inflammatory joint pain | JPR
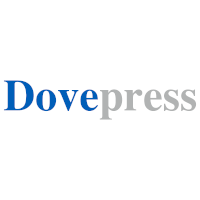
Introduction
The origins of pain are tightly linked to the expression of pro-inflammatory cytokines such as tumor necrosis factor (TNF), interleukin-6 (IL-6) and interleukin-1β (IL-1β) which can lead to neuronal hypersensitivity and increased pain states in patients.1,2 In many chronic inflammatory joint diseases such as osteoarthritis (OA) and gout, hyperactive immune cells within the joints cause increased expression of pro-inflammatory cytokines, leading to the further recruitment of inflammatory immune cells into the joint – ultimately causing progressive bone and cartilage degeneration.3,4 To this end, previous research has shown that continual expression of inflammatory cytokines can modulate nociceptor activity through intracellular changes in ERK and MAPK phosphorylation, leading to heightened nociceptor activity and spontaneous neuronal firing.5–7 In addition to immune cells, other cells within the joint such as chondrocytes and synoviocytes can modulate the inflammatory microenvironment that contributes to nociceptor sensitivity and the recruitment of other immune cells.8,9 Other mechanisms leading to the development of neuropathic pain include the degeneration of the joint anatomy over time, causing direct neuronal damage through pinching, nerve shearing and stretching, which can damage the nociceptor terminals and axons in the joint. The expression of inflammatory cytokines/chemokines and direct tissue damage act in a positive feed-forward mechanism leading to continual joint deterioration and cytokine expression.
In the context of OA and gout, a suite of inflammatory cytokines has been implicated in the etiology of joint pain. Notably, TNF is recognized as a key modulator of inflammatory responses and plays an integral role in the pathogenesis of these conditions. Recent studies have highlighted that TNF levels and those of other pro-inflammatory cytokines are significantly elevated in many chronic inflammatory diseases, including OA and gout.10,11 While no anti-TNF therapies to date have been approved to treat chronic inflammatory pain conditions, the IL-1β antagonist canakinumab has been approved for gout flares in adults who are contraindicated, intolerant to, or experience an inadequate response to NSAIDs.12 In OA, canakinumab has shown promising efficacy in slowing the progression of joint deterioration in these patients, leading to a decrease in the incidence of patients needing hip and knee replacements by 40% and 47%, respectively.13 This evidence indicates that the strategic inhibition of pro-inflammatory cytokines offers a logical and innovative method for formulating advanced therapies for chronic inflammatory diseases, including OA and gout.
For the treatment of OA and gout pain, prevailing clinical guidelines largely involve the administration of NSAIDs.14,15 These agents exert their anti-inflammatory action by curtailing the synthesis of prostaglandins, through the inhibition of cyclooxygenase enzymes, mainly COX-1 and COX-2.16–18 Suppression of prostaglandin production has been shown to not only modulate inflammation but also diminish pain perception by reducing nociceptor sensitivity to noxious stimuli.19,20 Emerging research indicates that NSAID therapy in OA may also downregulate the production of pivotal pro-inflammatory cytokines such as TNF. This has been shown through the modulation of signaling pathways including ERK, JNK, p38, and RAS, suggesting a potential immunomodulatory effect of NSAIDs that extends beyond their analgesic roles.21 However, NSAIDs are short-acting and fail to alleviate severe pain and ultimately many patients are frequently prescribed opioids for pain management.14,22,23 Despite their widespread use, opioids have shown limited efficacy in the long-term management of chronic pain and are associated with significant safety concerns, including high risks of respiratory depression, gastrointestinal constipation, addiction and overdose.22–25 These challenges have catalyzed a series of initiatives, both public and private, aimed at mitigating opioid misuse and encouraging the investigation of non-opioid analgesic alternatives.26,27 Therefore, it is imperative to further investigate the role of novel drug targets in the inflammatory processes and pain associated with chronic joint diseases like OA and gout.
Recent discovery of transforming growth factor-β-activated kinase 1 (TAK1) in the TNF signaling pathway has identified a novel target for the treatment of inflammatory joint-associated pain. TAK1 functions as a pivotal signaling hub within biochemical signaling pathways that regulate the production of various cytokines including TNF, IL-6 and IL-1β.28–30 When activated, TAK1 triggers the production of inflammatory cytokines and promotes pro-survival gene expression through NF-κβ.31 Recent data has identified that TAK1 plays a critical role in the development of chronic pain.32 Previous work has shown that TAK1 inhibition can block the expression of several key cytokines in the dorsal root ganglia and prevent the development of mechanical pain in three models of inflammatory, neuropathic and functional pain.32 Additionally, work in the central nervous system (CNS) has shown that TAK1 expression in astrocytes and microglia can contribute to CNS inflammation and the development of chronic pain, and that reduction of TAK1 expression in the CNS by antisense oligodeoxynucleotide intrathecal administration significantly prevented and reversed peripheral nerve injury.33–35
Despite this work validating TAK1 as a novel analgesic target, limitations of our earlier work stemmed from the use of takinib, which was a selective and low nM (IC50 = 9nM) inhibitor of TAK1 but lacked oral bioavailability.36 Recently, our group performed structure activity relationship studies that identified a promising analog of takinib, HS-276, which is a highly potent (IC50 = 2.5nM) and exquisitely selective (kinase selectivity score = 0.04) inhibitor of TAK1 with high oral bioavailability (%F = 98%).37 Here, we evaluate the ability of HS-276 to attenuate mechanical allodynia in the rat MSU gouty arthritis model as well as the rat MIA osteoarthritis model. Our work indicates that TAK1 inhibition with HS-276 attenuates mechanical allodynia and dynamic weight-bearing in male and female rats in the MIA model of OA, accompanied by a significant reduction in MIA-induced joint degeneration measured by knee histology. Furthermore, in the MSU model of gouty arthritis, TAK1 inhibition leads to a significant reduction in knee edema and mechanical allodynia in female rats but not male rats. These results suggest that our novel, potentially first-in-class, orally bioavailable anti-TNF therapeutic could provide a more effective and/or safer alternative to current joint-targeted pain treatments.
Methods
Animal Care
All animal experiments were approved and carried out in accordance with the Inotiv Boulder Institution Animal Care and Use Committee (IACUC; protocol number IB-040) and conformed to the National Institutes of Health Guide for the Care and Use of Laboratory Animals and the ARRIVE (Animal Research: Reporting of In Vivo Experiments) guidelines. IACUC approval was obtained by Inotiv Boulder (contract research organization) to comply with current animal care standards. Rats were fed at libitum with Harlan Teklad #8640 and tap water. Continual temperature and humidity were monitored in the animal housing and rats maintained on a 12hr/12hr light/dark cycle.
General Experimental Design for MSU Model
Upon arrival, animals were acclimated to the mechanical and thermal testing apparatus.
Prior to the study initiation, animals were allocated to groups and baseline caliper and behavior measurements were taken. On study day 0, the animals began treatment with HS-276 via oral gavage. i.a. injections of PBS or MSU were administered 1 hour after the first dose, and again on study day 1. Animal body weights were measured, and interim blood was collected. Knee caliper measurements were taken as described below. Von Frey, dynamic weight-bearing, and Hargreaves testing were performed as described below. Animals were necropsied after the final behavioral assessments (approximately 24h following the second i.a. injection). The evaluation of efficacy was based on knee caliper measurements, pain testing, weight-bearing, and knee histopathology.
Mechanical Von Frey Measurements
Von Frey analysis was conducted on study days −4 (baseline), −1, 0, 1, and 2 for the animals. Before enrollment, rats were acclimated to the animal colonies and familiarized with the testing rack through two to three sessions. Our Von Frey testing kit included hairs ranging from 3.16 to 5.18 grams, representing the absolute threshold for rats. To ensure consistency, each researcher utilized the same set of hairs, preventing variability between kits. Data was entered into a spreadsheet, and the PsychoFit program was employed to convert the 100% response rate into a 50% threshold for reporting results.
The testing procedure was initiated with three applications of the 4.31 hair. A recorded response indicated an observable reaction, such as lifting the hind paw from the grate to alleviate pressure. Responses were documented as either 0 (no response) or 1 (response) on a template. If the animal did not respond to the hair in three consecutive trials, the next larger hair in the kit was applied, and the process was reiterated until the animal responded three times consecutively. Following a response, the previous filament was re-tested to confirm the absence of response. Conversely, if the animal responded, the next smaller filament was applied and the process continued until the animal no longer responded. Once a lack of response was confirmed, the previous filament was retested to verify the response.
Testing specifically targeted the hind portions of the hind paw, as the heel was deemed to provide a more reliable and sensitive response. Testers observed animals for hyper-responding or freezing, in which case the animals were left undisturbed until they calmed down.
Knee Caliper Measurements
Caliper measurements were conducted employing a Mitutoyo Digimatic Micrometer Series 293. Initial knee caliper measurements were obtained from a single knee, and the values were rounded to one-thousandth (0.001) of a millimeter. To ascertain clinical normalcy, these measurements were cross-referenced with historical values for rats, taking into account various body weights. Subsequently, the baseline measurements were extended to encompass both knees, and these values were retained as long as the joint exhibited clinical normalcy without any signs of inflammation. The disparity between the right (injected) and left (non-injected) knees was then computed.
General Experimental Design for the MIA Model
Animals were weighed and randomized by body weight on study day −3 and underwent baseline electronic Von Frey and dynamic weight-bearing testing prior to study initiation. On study day 0, animals were anesthetized, their right knees shaved and prepped with 70% EtOH and then injected with saline (disease control) or 2 mg Mono-Iodo Acetate (Sigma Cat #: I2512-25G) into the right knee joint to induce disease. On study day 1, animals were dosed with either HS-276 30 or 50 mg/kg P.O. BID or vehicle (sterile water). Behavior assessment involving electronic Von Frey (eVF) and dynamic weight-bearing were performed by a blinded evaluator on days 4,7,14,21 and 28. After pain testing on day 28, animals were sacrificed by isoflurane anesthesia following a bilateral pneumothoracotomy.
Dynamic Weight-Bearing
Prior to testing, the force sensor is cleaned lightly with 70% isopropyl alcohol and wiped clean of any debris. The lid is placed on the dynamic weight-bearing chamber, and the camera was checked for proper alignment and adjusted as necessary. Rats were placed into the dynamic weight-bearing testing chamber (BioSeb Cat. #: BIO-DWB-DUAL) and allowed to move freely for 2 minutes. Approximately one minute of video was used for data analysis. Animals were assessed for the amount of weight placed on the left and right hind paws (in grams), and data reported as grams of difference between each foot.
Electric Von Frey Measurements
Rats were habituated to animal colonies for one week and handled four times for five minutes each after one week of habituation. Rats were habituated to the testing rack three times during this process. Rats were tested using an Almemo 2490 electronic Von Frey testing device. After a minimum of 5 minutes of acclimation, the instrument was zeroed out and then gentle pressure applied with the rigid tip to the testing area (between the foot pads/palm of the injected hind limb) at a 90° angle. Constant pressure was applied until the rat raised its foot in response to the stimulus. If it was unclear if the rat moved, rather than a true response, the rat was re-tested after the prescribed waiting period. Each rat was tested 3 times with a minimum of 8 minutes between testing events. The force applied for each response was averaged for each animal.
Cytokine Analysis
Baseline, interim, and terminal serum samples, along with synovial lavage fluid, were gathered from each group of animals and subjected to analysis using Luminex 200TM. The cytokines MIP-1α, IL-1β, IL-2, IL-6, IFNγ, and TNF were specifically targeted. The EMD Milliplex MAP Rat Cytokine/Chemokine Magnetic Panel (Kit catalogue No. RECYTMAG-65K, lot No. 3819884) was employed for cytokine analysis. To initiate the process, 200μL of wash buffer was introduced into each plate well, which was then sealed and mixed on a plate shaker for 10 minutes at room temperature (20–25°C). Following this, the wash buffer was decanted, and any residual amount was removed by inverting the plate and tapping it onto absorbent towels. Standard or control solutions (50 μL each) were added to the appropriate wells, with the serum matrix serving as the background for the 0 pg/mL standard. Subsequently, 25μL of assay buffer was added to the sample wells, and 25μL of the sample was introduced into the appropriate wells.
The plate was then sealed, wrapped in foil, and incubated with agitation on a plate shaker overnight (16–18 hours) at 2–8°C. After gently removing the well contents, the plate was washed three times following kit instructions. To continue the process, 25 μL of detection antibodies were added to each well, which was then sealed, covered with foil, and incubated with agitation on a plate shaker for 1 hour at room temperature (20–25°C). Subsequently, 25 μL of streptavidin-phycoerythrin was added to each well containing the detection antibodies. The wells were once again sealed, covered with foil, and incubated with agitation on a plate shaker for 30 minutes at room temperature (20–25°C). After gently removing the well contents, the plate underwent three washes according to kit instructions.
Following this, 150 μL of Sheath Fluid was added to all wells, and the beads were resuspended on a plate shaker for 5 minutes. The plate was then run on Luminex 200TM, and the absolute expression was determined by a standard curve of known analyte expression. The data were expressed as cytokine concentration (pg/mL).
Histology
The knees that received injections from all animals were harvested, had their skin removed, were trimmed of muscle, and then preserved in 10% neutral buffered formalin (NBF) for histological examination. The injected knees were bisected into approximately equal halves along the frontal plane and subsequently embedded in paraffin. From each paraffin-embedded block, sections were cut and stained with toluidine blue. Microscopic analysis was conducted on the tissues, with scoring performed for inflammation, pannus formation, cartilage damage, and bone resorption. The individual scores for these histopathological features were then summed to calculate a comprehensive histopathology score for each joint.
Scoring Criteria
The following scoring system was used to evaluate the histopathological changes observed in the joints.
Inflammation Score: 0 = normal; 0.5 = very minimal, generally focal or multifocal very minor inflammation; 1 = minimal, multifocal, minor infiltration of inflammatory cells in
synovium/periarticular tissue, no obvious distension of joint capsule; 2 = mild infiltration, diffuse mild infiltration of entire synovium, minor if any distension of joint capsule; 3 = moderate infiltration with moderate edema, diffuse with moderate distension of joint capsule usually on both medial and lateral sides; 4 = marked infiltration with marked edema, diffuse with marked distension of joint capsule on both medial and lateral sides or may have just one side severely distended and the other is milder; 5 = severe infiltration with severe edema, diffuse with severe distension of joint capsule on both medial and lateral sides; 6 = very severe infiltration with very severe edema, diffuse with very severe distention of joint capsule on both medial and lateral sides.
Pannus Score: 0 = normal; 0.5 = minimal infiltration of pannus in cartilage and subchondral bone, affects only marginal zones and affects only a few focal areas, less than 1% of area at risk
Affected; 1 = minimal infiltration of pannus in cartilage and subchondral bone, approximately 1–10% of cartilage surface or subchondral bone affected; 2 = mild infiltration (extends over up to 1/4 of surface or subchondral area of tibia or femur), approximately 11–25% of cartilage surface or subchondral bone affected, 3 = moderate infiltration (extends over >1/4 but
Cartilage Damage Score: 0 = normal; 0.5 = minimal decrease in toluidine blue staining, affects only marginal zones; 1 = minimal to mild loss of toluidine blue staining with less than 1%
cartilage loss on all combined surfaces; 2 = mild loss of toluidine blue staining with focal mild (superficial) chondrocyte loss and/or collagen disruption, may have few small areas
of 50% depth of cartilage affected, 1–10% cartilage loss on all combined surfaces; 3 = moderate loss of toluidine blue staining with multifocal to diffuse moderate (depth to middle zone) chondrocyte loss and/or collagen disruption, may have several areas of full thickness loss, 11–25% cartilage loss on all combined surfaces; 4 = marked loss of toluidine blue staining with multifocal to diffuse marked (depth to deep zone) chondrocyte loss and/or collagen
disruption, total overall loss 26–50% of all combined surfaces; 5 = severe diffuse loss of toluidine blue staining with multifocal severe (depth to tide mark), total overall loss greater than 50% of width of all surfaces combined.
Bone Resorption Score: 0 = normal; 0.5 = minimal resorption affects only marginal zones, less than 1% of area at risk affected; 1 = small areas of resorption, not readily apparent on low magnification, approximately 1–10% of total joint width of subchondral bone affected; 2 = more numerous areas of resorption, definite loss of subchondral bone, approximately 11–25% of total joint width of subchondral bone affected; 3 = obvious resorption of subchondral bone approximately 26–50% of total joint width of subchondral bone affected; 4 = obvious resorption of subchondral bone approximately 51–75% of total joint width of subchondral bone affected; 5 = distortion of entire joint due to destruction approximately 76–100% of total joint width of subchondral bone affected.
Osteophyte Measurement and Score
Osteophyte expansion in the marginal zones was assessed utilizing an ocular micrometer. The area with the most extensive proliferation (considered the worst-case scenario) was quantified and subsequently evaluated based on the following criteria: Grade 0 = None, Grade 1 = 200–399μm, Grade 2 = 400–599μm, Grade 3=600-799μm, Grade 4 = 800–999 μm, Grade 5 ≥ 1000μm.
Statistical Analysis
GraphPad Prism 9 software was used for all statistical tests and graph presentations. Statistical tests for each assay are mentioned in the figure legends.
Results
We evaluated the anti-inflammatory and analgesic effects of TAK1 inhibition by HS-276 in both the MIA model of osteoarthritis and MSU model of gouty arthritis. In the MIA model, male and female rats developed prominent hypersensitivity to mechanical stimuli (Von Frey filaments) on the ipsilateral hind paw persisting for 28 days post-monoiodoacetate intra-articular injection (naïve vs vehicle comparison; males p = 0.001, females p = 0.009) (Figure 1a and b). Both male and female rats treated with HS-276 at 50 mg/kg P.O. BID showed significant attenuation of monoiodoacetate-induced mechanical hyperalgesia compared to vehicle-treated rats (males F3,149=75.28 p 3,92=35.07 p Figure 1a and b).
Clinically, OA often presents with non-symmetric joint degeneration between the left and right knees leading to changes in a patient’s gait.38,39 This compensation can often be directly related to the pain severity reported in the joint, leading patients to avoid placing pressure on the affected joint. Here, we analyzed the changes in weight-bearing between the OA injured (right) and non-injured (left) hind legs. Analysis of dynamic weight-bearing in male and female rats showed that intra-articular injection of MIA in rats treated with vehicle (water) significantly increased the differential in weight-bearing between injured (right) and non-injured (left) hind legs compared to naïve animals (males p Figure 1c and d). Treatment with HS-276 reduced the differential weight-bearing score in both 30 and 50 mg/kg treated groups for both males (p = 0.0012, p Figure 1c and d). Throughout the study, both male and female rats showed no significant or dose-dependent weight loss (Supplemental Figure 1a and b).
We have previously seen that TAK1 inhibition can block the infiltration of immune cells into the affected joint(s) in models of rheumatoid arthritis.37,40 We next sought to explore the pathological effects of TAK1 inhibition on the MIA-treated knees at the study terminus (Day 28). Vehicle-treated animals showed histopathological changes consistent with the MIA model, including increased synovial inflammation, fibrosis, bone resorption and osteophyte formation (Figure 2a). Evaluation of histological scores in males showed HS-276 treated animals exhibited significantly reduced synovial inflammation (45%, p = 0.009), synovial fibrosis (39%, p = 0.001), osteophyte score (46%, p=0.03), and osteophyte width (28%) (Figure 2b). Similarly, female HS-276 treated animals showed a significant reduction in synovial fibrosis (55%, p = 0.008), bone and cartilage resorption (50%, p Figure 2c).
We next sought to validate the analgesic effects of HS-276 in another model of joint pain – gouty arthritis. Gouty arthritis is characterized by the buildup of uric acid crystals in the joints leading to a chronic inflammatory state and continual joint degeneration.41 In this model, male and female rats received i.a. injections of monosodium urate on days 1 and 3. Knee edema was measured 2 hours post-MSU injection in vehicle, gabapentin and HS-276 treated groups. Female HS-276 (50 mg/kg) treated animals showed a significant reduction in knee edema 2 hours post-2nd MSU i.a. injection (p = 0.003), with no significant reduction in edema observed in gabapentin (150 mg/kg) treated animals (p = 0.12) (Figure 3a). Male rats showed less edema overall following both 1st and 2nd MSU injections, with no significant reduction in knee edema observed in HS-276 or gabapentin-treated animals (Figure 3b). Evaluation of mechanical allodynia in female rats showed HS-276 significantly attenuated mechanical allodynia, measured by Von Frey filaments (p = 0.013) (Figure 3c). Male rats showed a similar trend of reduced mechanical allodynia in HS-276 treatment groups, however this was not statistically significant (Figure 3d).
Morphological evolution of the knees from naïve and MSU-treated animals showed MSU i.a. injections significantly increased inflammation that ranged from minimal to severe as well as significant cartilage damage scores (Figure 4a–c). Treatment with HS-276 significantly reduced the mean Histology score in female (p = 0.01; Figure 4b) but not male (p = 0.79; Figure 4d) rats. Furthermore, serum analysis from vehicle, HS-276 and gabapentin-treated animals showed that female rats had decreased TNF and IL-1β serum cytokine expression (Supplemental Figure 2).
Discussion
Identifying novel drug targets for the treatment of chronic joint pain such as OA and gout is essential to developing safer and more effective treatment options. Here, we explored the therapeutic potential of selective TAK1 inhibition with the small molecule TAK1 inhibitor, HS-276, in modulating joint pain in two distinct rodent models of OA (MIA) and gout (MSU). In our OA studies, we employed the MIA model, which has been shown to induce a significant pro-inflammatory microenvironment in the joints.42–44 Compared to other models such as the destabilization of the medial meniscus (DMM), the induction of inflammation through selective chondrocyte depletion by MIA creates an inflammatory phenotype in the knee leading to neovascularization and subchondral bone necrosis. As a known inflammatory target, studying TAK1 in this model demonstrates the immune modulatory and analgesic potential of TAK1 inhibition. Future studies exploring the efficacy of TAK1 inhibition in joint degeneration and analgesia in the DMM model will be conducted to identify overlapping mechanisms of analgesia between both an inflammatory and traumatic injury model. In these studies, using OA and gouty arthritis models, we showed that HS-276 significantly blocked disease development and provided analgesic relief in animals receiving treatment. Furthermore, we showed that HS-276 treatment was able to block histological deterioration of the joints induced by disease onset.
Joint degeneration and subsequent pain in the knees often advances non-symmetrically, leading to patients developing gait issues. These changes in gait contribute to both structural changes in the joint such as changes in the collagenous networks in the posterior meniscal horn, as well as thickening of fibers within the superficial and deep zones of the articular cartilage leading to tissue degradation as well as neuronal sensitivity, causing patients to avoid excessive weight-bearing on an injured knee.45,46 Our work herein has identified that TAK1 inhibition significantly blocked MIA-induced joint degeneration leading to a significant prevention of differential weight-bearing in HS-276 treated groups. Prevention or slowing of knee degeneration is expected to impact the development of chronic pain in patients, either delaying its onset or reducing the pain levels reported. As the knee joint continues to lose cartilage and bone stability, primary nerve afferents innervating the knee become hypersensitized by inflammatory mediators present. In addition to hyper-nociceptor activity induced by aberrant inflammatory signaling, severe joint degeneration can cause nerve entrapment, crushing and die back due to continual mechanical pressure in the joint. Data provided herein and previously by our group support that TAK1 sits at the crossroads of both these processes and can prevent disease progression as well as limit the expression of inflammatory cytokines.
A primary hypothesis of our work is that TAK1 inhibition in part works by blocking the expression of several key inflammatory cytokines that regulate joint degeneration and pain states. While our data supported that treatment with HS-276 reduced the expression of these cytokines, the data were not significant. There can be many reasons for this result, for example, our group has previously seen that measuring cytokines in pre-clinical models of disease can have varied expression patterns during the acute and chronic stages of disease. Often the expression of inflammatory mediators in the acute stage is greater than in the chronic stage (where we sampled cytokines), and thus it is plausible we missed the greatest expression time of cytokines. Furthermore, because these are targeted disease inductions via i.a. injections, systemic cytokine measurements from serum may not be the best surrogate to understand the expression levels at the site of active diseases. Future sampling of synovial knee fluid from both the injured and non-injured knees may elucidate greater tissue site-specific changes in cytokines as a result of HS-276 treatment.
Current treatments for OA and gout aim to alleviate pain and improve joint function, which ranges from non-pharmacological approaches such as exercise, weight loss and other lifestyle changes to pharmacologic interventions depending on pain intensity.47,48 While many treatments are effective, all carry a variety of shortcomings including both marginal efficacy and/or significant safety risks. Patients who report chronic pain are often prescribed NSAIDs and opioid treatments, the latter of which carry long-term safety concerns including addiction and overdose risks due to misuse.49 Currently, there is a large unmet need to develop new drug targets for the treatment of OA that can adequately prevent and reduce the chronic pain observed in many patients. Thus, patients with chronic pain from OA continue to suffer severe physiological and psychological outcomes, with current treatment options showing limited clinical benefit over the long term, especially for chronic opioid users. Recent work on TAK1 has further elucidated its role in TNF signaling as well as autoimmune diseases, providing greater context to the potential therapeutic effects of this target.31,50 The development of a novel, highly selective and potent, orally bioavailable inhibitor of TAK1 could provide OA and gout pain patients a safer and more effective alternative to current treatment options.
Acknowledgments
We would like to thank Bolder BioPath (Inotiv) for performing the in vivo MIA and MSU models.
Funding
This work was funded by the National Institutes of Health’s (NIH) National Institute of Neurological Disorders and Stroke (NINDS) grant R43NS119087-01A1 to S.S and T.H.
Disclosure
Robert Freeze, Phillip Hughes, Timothy Haystead and Scott Scarneo are all shareholders of EydisBio. The funding sources did not play a role in shaping the experimental design, influencing data analysis, or contributing to the preparation of the manuscript. In addition, Philip Hughes has a patent US10207998B2 licensed to EydisBio, a patent US10927083B2 licensed to EydisBio, a patent WO2022164822 licensed to EydisBio; and Philip Hughes is a founder and part owner of EydisBio, where this work was done. Timothy Haystead reports personal fees from EydisBio, outside the submitted work. In addition, Dr Timothy Haystead has a patent DU7027US | US App. No. 18/273,843 pending at Duke University. The authors report no other conflicts of interest in this work.
References
1. Sommer C, Kress M. Recent findings on how proinflammatory cytokines cause pain: peripheral mechanisms in inflammatory and neuropathic hyperalgesia. Neurosci Lett. 2004;361(1–3):184–187. doi:10.1016/j.neulet.2003.12.007
2. Vanderwall AG, Milligan ED. Cytokines in pain: harnessing endogenous anti-inflammatory signaling for improved pain management. Front Immunol. 2019;10:3009. doi:10.3389/fimmu.2019.03009
3. Lopes EBP, Filiberti A, Husain SA, Humphrey MB. Immune contributions to osteoarthritis. Curr Osteoporos Rep. 2017;15(6):593–600. doi:10.1007/s11914-017-0411-y
4. de Lange-Brokaar BJ, Ioan-Facsinay A, van Osch GJVM, et al. Synovial inflammation, immune cells and their cytokines in osteoarthritis: a review. Osteoarthritis Cartilage. 2012;20(12):1484–1499. doi:10.1016/j.joca.2012.08.027
5. Wieseler-Frank J, Maier SF, Watkins LR. Central proinflammatory cytokines and pain enhancement. Neurosignals. 2005;14:166–174. doi:10.1159/000087655
6. Matsuda M, Huh Y, Ji RR. Roles of inflammation, neurogenic inflammation, and neuroinflammation in pain. J Anesth. 2019;33(1):131–139. doi:10.1007/s00540-018-2579-4
7. Obata K, Noguchi K. MAPK activation in nociceptive neurons and pain hypersensitivity. Life Sci. 2004;74(21):2643–2653. doi:10.1016/j.lfs.2004.01.007
8. Arra M, Abu-Amer Y. Cross-talk of inflammation and chondrocyte intracellular metabolism in osteoarthritis. Osteoarthritis Cartilage. 2023;31(8):1012–1021. doi:10.1016/j.joca.2023.04.003
9. Konttinen YT, Sillat T, Barreto G, Ainola M, Nordstrom DC. Osteoarthritis as an autoinflammatory disease caused by chondrocyte-mediated inflammatory responses. Arthritis Rheum. 2012;64(3):613–616. doi:10.1002/art.33451
10. Kapoor M, Martel-Pelletier J, Lajeunesse D, Pelletier JP, Fahmi H. Role of proinflammatory cytokines in the pathophysiology of osteoarthritis. Nat Rev Rheumatol. 2011;7(1):33–42. doi:10.1038/nrrheum.2010.196
11. Shi M, Luo J, Ding L, Duan L. Spontaneous resolution of acute gout: mechanisms and therapeutic targets. RMD Open. 2023;9(3):e003586. doi:10.1136/rmdopen-2023-003586
12. ILARIS® (canakinumab) injection [Package Insert], East Hanover, NJ: Novartis, 2009.
13. Schieker M, Conaghan PG, Mindeholm L, et al. Effects of interleukin-1beta inhibition on incident hip and knee replacement: exploratory analyses from a randomized, double-blind, placebo-controlled trial. Ann Intern Med. 2020;173(7):509–515. doi:10.7326/M20-0527
14. Kolasinski SL, Neogi T, Hochberg MC, et al. 2019 American college of rheumatology/arthritis foundation guideline for the management of osteoarthritis of the hand, hip, and knee. Arthritis Rheumatol. 2020;72(2):220–233. doi:10.1002/art.41142
15. FitzGerald JD, Dalbeth N, Mikuls T, et al. 2020 American College of rheumatology guideline for the management of gout. Arthritis Care Res. 2020;72(6):744–760. doi:10.1002/acr.24180
16. Rao P, Knaus EE. Evolution of nonsteroidal anti-inflammatory drugs (NSAIDs): cyclooxygenase (COX) inhibition and beyond. J Pharm Pharm Sci. 2008;11(2):81s–110s. doi:10.18433/J3T886
17. Romsing J, Moiniche S. A systematic review of COX-2 inhibitors compared with traditional NSAIDs, or different COX-2 inhibitors for post-operative pain. Acta Anaesthesiol Scand. 2004;48(5):525–546. doi:10.1111/j.0001-5172.2004.00379.x
18. Crofford LJ. Use of NSAIDs in treating patients with arthritis. Arthritis Res Ther. 2013;15(Suppl S3):2. doi:10.1186/ar4174
19. Jang Y, Kim M, Hwang SW. Molecular mechanisms underlying the actions of arachidonic acid-derived prostaglandins on peripheral nociception. J Neuroinflammation. 2020;17(1):30. doi:10.1186/s12974-020-1703-1
20. Burian M, Geisslinger G. COX-dependent mechanisms involved in the antinociceptive action of NSAIDs at central and peripheral sites. Pharmacol Ther. 2005;107(2):139–154. doi:10.1016/j.pharmthera.2005.02.004
21. Gallelli L, Galasso O, Falcone D, et al. The effects of nonsteroidal anti-inflammatory drugs on clinical outcomes, synovial fluid cytokine concentration and signal transduction pathways in knee osteoarthritis. A randomized open label trial. Osteoarthritis Cartilage. 2013;21(9):1400–1408. doi:10.1016/j.joca.2013.06.026
22. Machado GC, Abdel-Shaheed C, Underwood M, Day RO. Non-steroidal anti-inflammatory drugs (NSAIDs) for musculoskeletal pain. BMJ. 2021;372:n104. doi:10.1136/bmj.n104
23. da Costa BR, Pereira TV, Saadat P, et al. Effectiveness and safety of non-steroidal anti-inflammatory drugs and opioid treatment for knee and Hip osteoarthritis: network meta-analysis. BMJ. 2021;375:n2321. doi:10.1136/bmj.n2321
24. van den Driest JJ, Schiphof D, de Wilde M, et al. Opioid prescriptions in patients with osteoarthritis: a population-based cohort study. Rheumatology. 2020;59(9):2462–2470. doi:10.1093/rheumatology/kez646
25. Nadeau SE, Wu JK, Lawhern RA. Opioids and chronic pain: an analytic review of the clinical evidence. Front Pain Res. 2021;2:721357. doi:10.3389/fpain.2021.721357
26. Helmerhorst GT, Teunis T, Janssen SJ, Ring D. An epidemic of the use, misuse and overdose of opioids and deaths due to overdose, in the United States and Canada: is Europe next? Bone Joint J. 2017;99(7):856–864. doi:10.1302/0301-620X.99B7.BJJ-2016-1350.R1
27. Koh HK. Community-based prevention and strategies for the opioid crisis. JAMA. 2017;318(11):993–994. doi:10.1001/jama.2017.13767
28. Scarneo SA, Mansourati A, Eibschutz LS, et al. Genetic and pharmacological validation of TAK1 inhibition in macrophages as a therapeutic strategy to effectively inhibit TNF secretion. Sci Rep. 2018;8(1):17058. doi:10.1038/s41598-018-35189-7
29. Scarneo SA, Eibschutz LS, Bendele PJ, et al. Pharmacological inhibition of TAK1, with the selective inhibitor takinib, alleviates clinical manifestation of arthritis in CIA mice. Arthritis Res Ther. 2019;21(1):292. doi:10.1186/s13075-019-2073-x
30. Scarneo SA, Hughes PF, Yang KW, et al. A highly selective inhibitor of interleukin-1 receptor-associated kinases 1/4 (IRAK-1/4) delineates the distinct signaling roles of IRAK-1/4 and the TAK1 kinase. J Biol Chem. 2020;295(6):1565–1574. doi:10.1074/jbc.RA119.011857
31. Totzke J, Scarneo SA, Yang KW, Haystead TAJ. TAK1: a potent tumour necrosis factor inhibitor for the treatment of inflammatory diseases. Open Biol. 2020;10(9):200099. doi:10.1098/rsob.200099
32. Scarneo S, Zhang X, Wang Y, et al. Transforming Growth Factor-beta-Activated Kinase 1 (TAK1) mediates chronic pain and cytokine production in mouse models of inflammatory, neuropathic, and primary pain. J Pain. 2023;24(9):1633–1644. doi:10.1016/j.jpain.2023.04.011
33. Katsura H, Obata K, Miyoshi K, et al. Transforming growth factor-activated kinase 1 induced in spinal astrocytes contributes to mechanical hypersensitivity after nerve injury. Glia. 2008;56(7):723–733. doi:10.1002/glia.20648
34. Goldmann T, Wieghofer P, Müller PF, et al. A new type of microglia gene targeting shows TAK1 to be pivotal in CNS autoimmune inflammation. Nat Neurosci. 2013;16(11):1618–1626. doi:10.1038/nn.3531
35. Soto-Diaz K, Juda MB, Blackmore S, Walsh C, Steelman AJ. TAK1 inhibition in mouse astrocyte cultures ameliorates cytokine-induced chemokine production and neutrophil migration. J Neurochem. 2020;152(6):697–709. doi:10.1111/jnc.14930
36. Totzke J, Gurbani D, Raphemot R, et al. Takinib, a selective TAK1 inhibitor, broadens the therapeutic efficacy of TNF-alpha inhibition for cancer and autoimmune disease. Cell Chem Biol. 2017;24(8):1029–1039e1027. doi:10.1016/j.chembiol.2017.07.011
37. Scarneo S, Hughes P, Freeze R, et al. Development and efficacy of an orally bioavailable selective TAK1 inhibitor for the treatment of inflammatory arthritis. ACS Chem Biol. 2022;17(3):536–544. doi:10.1021/acschembio.1c00788
38. Christiansen CL, Stevens-Lapsley JE. Weight-bearing asymmetry in relation to measures of impairment and functional mobility for people with knee osteoarthritis. Arch Phys Med Rehabil. 2010;91(10):1524–1528. doi:10.1016/j.apmr.2010.07.009
39. Garsden LR, Bullock-Saxton JE. Joint reposition sense in subjects with unilateral osteoarthritis of the knee. Clin Rehabil. 1999;13(2):148–155. doi:10.1191/026921599674996411
40. Freeze R, Yang KW, Haystead T, Hughes P, Scarneo S. Delineation of the distinct inflammatory signaling roles of TAK1 and JAK1/3 in the CIA model of rheumatoid arthritis. Pharmacol Res Perspect. 2023;11(4):e01124. doi:10.1002/prp2.1124
41. Schlesinger N. Treatment of chronic gouty arthritis: it is not just about urate-lowering therapy. Semin Arthritis Rheum. 2012;42(2):155–165. doi:10.1016/j.semarthrit.2012.03.010
42. Pitcher T, Sousa-Valente J, Malcangio M. The monoiodoacetate model of osteoarthritis pain in the mouse. J Vis Exp. 2016;(111). doi:10.3791/53746-v
43. Zheng S, Ren J, Gong S, Qiao F, He J. CTRP9 protects against MIA-induced inflammation and knee cartilage damage by deactivating the MAPK/NF-kappaB pathway in rats with osteoarthritis. Open Life Sci. 2020;15(1):971–980. doi:10.1515/biol-2020-0105
44. Liu P, Okun A, Ren J, et al. Ongoing pain in the MIA model of osteoarthritis. Neurosci Lett. 2011;493(3):72–75. doi:10.1016/j.neulet.2011.01.027
45. Yoshioka NK, Young GM, Khajuria DK, et al. Structural changes in the collagen network of joint tissues in late stages of murine OA. Sci Rep. 2022;12(1):9159. doi:10.1038/s41598-022-13062-y
46. Aoyagi K, Liew JW, Farrar JT, et al. Does weight-bearing versus non-weight-bearing pain reflect different pain mechanisms in knee osteoarthritis?: the Multicenter Osteoarthritis Study (MOST). Osteoarthritis Cartilage. 2022;30(4):545–550. doi:10.1016/j.joca.2021.10.014
47. Holden MA, Metcalf B, Lawford BJ, et al.; An international consensus study from the OARSI Rehabilitation Discussion Group. Recommendations for the delivery of therapeutic exercise for people with knee and/or Hip osteoarthritis. Osteoarthritis Cartilage. 2023;31(3):386–396. doi:10.1016/j.joca.2022.10.009
48. Bennell KL, Lawford BJ, Keating C, et al. Comparing video-based, telehealth-delivered exercise and weight loss programs with online education on outcomes of knee osteoarthritis: a randomized trial. Ann Intern Med. 2022;175(2):198–209. doi:10.7326/M21-2388
49. Biancuzzi H, Dal Mas F, Brescia V, et al. Opioid misuse: a review of the main issues, challenges, and strategies. Int J Environ Res Public Health. 2022;19(18):11754. doi:10.3390/ijerph191811754
50. Bale S, Verma P, Yalavarthi B, et al. Pharmacological inhibition of TAK1 prevents and induces regression of experimental organ fibrosis. JCI Insight. 2023;8(14). doi:10.1172/jci.insight.165358